The role of parenteral nutrition in paediatric critical care, and its consequences on recovery
Introduction
Providing optimal nutrition is essential for normal growth, health and development of children. Nutrition is known to both cure and cause diseases, and with this viewpoint in mind, the role of parenteral nutrition (PN) has developed substantially over de last decade. During critical illness the child is subjected to hormonal and metabolic changes, commonly referred to as acute stress response, which temporarily inhibits the normal developmental process in order to survive. Furthermore, the gut is subjected to many adverse influences such as ischemia, altered blood flow, lack of enteral nutrition (EN) and medication. As such, the goal of nutritional support is to provide the appropriate amount of feeding during the different phases of disease in order to accelerate recovery and to have beneficial effects on short-term and long-term outcome. Nutritional requirements of critically ill children depend on many factors, including nutritional status on admission, the underlying and actual diagnosis. Furthermore, the awareness of the changes in amino acid, lipid, carbohydrate and micronutrient metabolism during the different phase of the acute stress response is essential in determining the dynamic metabolic and nutritional support, and thereby counteract malnourishment and overfeeding (Table 1).
Table 1
Phase | Definition | Nutritional considerations |
---|---|---|
Acute phase (catabolic) | First phase after event, characterized by requirement of (escalating) vital organ support. Phase when the patient requires vital organ support (sedation, mechanical ventilation, vasopressors, fluid resuscitation) | Energy acquired via endogenous production. Intake requirement lower than REE |
Start of enteral nutrition and accepting low and slowly inclining intakes up to 1 times REE, while monitoring patients EN tolerance | ||
Withhold PN during the acute phase (first 7 days) to allow autophagy and improve clinical outcomes | ||
Be aware of hypo- and hyperglycaemia | ||
Stable phase (catabolic – anabolic) | Stabilisation or weaning of vital organ support, while the different aspects of the stress response are not (completely) resolved. The patient is stable on, or can be weaned, from this vital support | Stepwise inclining EN intakes, while monitoring patients EN tolerance |
Provide PN from day 8 onwards | ||
Be aware of hyperglycaemia and IFALD | ||
Recovery phase (anabolic) | Clinical mobilisation with normalisation of neuro-endocrine, immunologic and metabolic alterations, characterized by a patient who is mobilizing | Higher caloric and protein requirements with EN and/or additional PN might be necessary to account for increasing physical activity, tissue repair, and long-term development |
EN, enteral nutrition; PN, parenteral nutrition; REE, resting energy requirement; IFALD, intestinal failure associated liver disease.
Nutritional support
The preferred route to provide nutritional support during paediatric critical illness is via enteral route (2-5). EN or even trophic feeding is supposed to have a positive influence on reduction of oxidative stress and maintaining the immune response and gastrointestinal mucosal integrity limiting bacterial translocation via the gut. However, the clinical impact of these positive modulations of EN is unknown. Due to many reasons, such as (perceived) feeding intolerance, fluid restriction, fasting around (bedside) procedures, target caloric and protein goals are often not achieved via enteral route and discrepancies between the amounts prescribed and delivered ranges up to 60% (6-11).
Observational studies have found that malnourishment and underfeeding due to macronutrient deficits are associated with delayed wound healing, reduced immune response, malabsorption, bacterial overgrowth and increased morbidity and mortality (8,9,12,13). Overfeeding in its turn may lead to intestinal failure associated liver disease (IFALD), hyperglycaemia and increased respiratory burden due to the increase in CO2 production present by lipogenesis from carbohydrates (14,15). Besides short-term consequences, both underfeeding and overfeeding have been associated with impaired growth, cognitive functioning and emotional and behavioural problems in non-critically ill children (16,17). Thus far, the long-term consequences of underfeeding and overfeeding in critically ill children have not been established.
Due to the inability to achieve caloric and protein goals via EN, PN is often initiated in critically ill children. A world-wide survey investigating PN practises in the paediatric intensive care units (PICUs) showed a wide variety between macronutrient and caloric targets, as well as estimation of energy requirements during critical illness and timing of initiation, amount and composition of PN (18,19). What should be considered as optimal PN during critical illness is controversial due to the majority of findings that are being derived from observational studies and the inability to provide a causal relationship between nutrition and short-term or long-term recovery and outcomes. To understand the optimal role of PN during paediatric critical illness, the following two fundamental questions should be answered:
- What is the optimal timing of PN?
- What is the optimal dose and composition of PN?
Timing of PN
Acute phase
The paediatric early versus late PN in critically ill children (PEPaNIC) RCT, published in 2016 was the first randomized controlled trial (RCT) that aimed to determine optimal timing in critically ill children (20). This large multicentre RCT involving 1,440 critically ill children showed that withholding supplemental PN for 7 days (Late PN), as compared with initiating PN within 24 hours after admission (early PN), improved short-term outcome such as new acquired infections and length of stay (20). EN was provided in both groups when possible and tolerated within 24 hours and PN was supplemented up to total caloric need following the randomisation groups. When more than 80% of total caloric need was reached enterally, supplemental PN was stopped. Weight deterioration during PICU admission was not affected by the intervention, however, a decrease in weight-for-age z-score itself was associated with worse clinical outcomes in both groups (21). Furthermore, secondary analyses of the PEPaNIC RCT showed that even term neonates and undernourished children upon admissions benefited from this intervention (22,23). The results of the PEPaNIC RCT had a great impact on international guidelines which currently advise to consider (supplemental) PN beyond day 7 of critical illness while providing micronutrients (2-5). So far, it is still the only RCT focusing on optimal initiation of PN in critically ill children in the first week of admission in the PICU.
Stable and recovery phase
Although restriction of PN during the acute phase of illness, continuing this course beyond the acute phase seems detrimental for short-term and long-term outcome. Currently, it is not known at which point in time safe parenteral restriction ends and the potential detrimental effects of macronutrient starvation starts.
During the stable and recovery phases, PN should focus on allowing normal or even catch up growth and successful provision is usually monitored through anthropometric measurements, muscle strength and function and tissue repair (e.g., wound healing). Nutritional needs can rise above normal requirements for healthy children (24), however, it is unclear how fast optimal feeding goals can be achieved. A stepwise caloric enhancement is recommended while providing EN, however, the guidelines do not provide recommendations for stepwise enhancement of PN (4).
Autophagy
The leading explanation behind the counter-intuitive finding of the PEPaNIC RCT is the consequence of early and high nutritional intake to suppress the fasting response, which induces ketosis and activates autophagy (25-28). Autophagy is an evolutionary conserved intracellular degradation process and it is crucial for maintaining cellular integrity and function. This becomes even more important during acute stress, as children suffer from extensive cell and organ damage, leading to organ failure and muscle weakness. Animal studies showed that impaired autophagic control caused by early PN let to liver and skeletal muscle deficiency (27). This process was confirmed by a study in adults establishing that early PN did not prevent muscle wasting and even increased adipose tissue deposition in the muscle (25). These studies open perspectives for therapies that activate autophagy during critical illness. Although still controversial, possible endeavours can lie within pharmacological agents inducing autophagy. For instance, an animal experiment found that stimulation of autophagy in the kidney with rapamycin correlated with protection of renal function (29).
Intermittent PN
PN can be provided continuously over 24 hours as well as intermittently, meaning a period of withholding PN. Several intermittent techniques have been described, including cyclic feeding with a period of fasting (10–12 hours) throughout the night or day. A cyclic regime in non-critically ill children with long-term PN, i.e., children with short bowel syndrome or intestinal failure, has been used for many years and was shown not to change the intestinal microbiome (30) and decreased the risk of IFALD and cholestasis (31). Furthermore, a reduction of serum bilirubin levels and livers enzymes was seen, which was associated with a reduction in both hyperinsulinaemia and fat deposition in the liver (32,33). Metabolic studies showed that lipid oxidation was higher and dextrose use was lower during cyclic PN (34,35). Overall, cyclic PN was well tolerated without a higher risk for hypo- or hyperglycaemia, however, using a tapering technique can be considered in younger children as abrupt discontinuation had may cause hypoglycaemia (36). Based on this evidence cyclic PN is currently recommended in stable patients during and after hospital admission (37).
Also, there is currently no evidence for continuous versus cyclic PN in critically ill children. Cyclic feeding has some additional hypothetical benefits in critical ill children compared to continuous provision of nutrients, e.g., fasting induces activation of autophagy, preservation of the circadian rhythm and even enhanced protein synthesis (28,38). This strategy remains controversial, however, the findings in the non-critically ill paediatric population underpin the rationale for a cyclic feeding strategy opposed of continuous feeding which is standard of care in most PICUs and opens perspectives for intervention studies in critically ill children to define an optimal fasting period to allow autophagy and potentially improve clinical outcomes.
Parenteral micronutrients
Micronutrients, consisting of vitamins, trace elements and electrolytes, are considered to have an important role in body metabolism, immune response and tissue function, and are therefore essential during critical illness. While the current guidelines on PN in critically ill children recommended to consider withholding PN for the first week of admission, they advise to maintain supplementation of micronutrients during this time window (2-5). In addition, the ESPGHAN/ESPEN/ESPR/CSPEN guidelines recommend to provide micronutrients daily because this prohibits adverse reactions from transient high levels, except from vitamin K which can be provided weekly without harmful side effects (39-41).
Dose of parenteral macronutrients
Energy
The actual energy requirement of the child will depend on many factors including medication, need for mechanical ventilation, temperature, (lack of) physical activity and on the phase of the disease. During the acute phase, endogenous energy production accounts for a substantial proportion of energy requirement (up to 75%) irrespective of the energy provision via exogenous source (42). Therefore, the energy requirement from EN or PN can be much lower than the calculated or measured resting energy expenditure (REE) (Figure 1). During the recovery phase the focus shifts from acute interventions to optimizing activity, tissue repair and physical and neurocognitive development. There is an increasing demand in energy during this phase to allow normal development of the child and even to catch up (2,4,43).
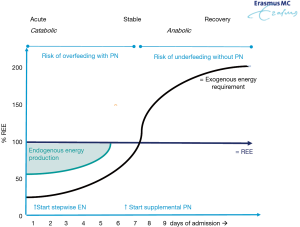
Amino acids
Amino acid dose requirement is lower via PN than EN due to the bypass of the utilization by the gastro-intestinal tract. A secondary analysis from the PEPaNIC study showed that during the acute phase higher doses of parenteral administered amino acids was negatively associated with PICU length of stay, new acquired infections and duration of mechanical ventilation (44). Even low doses of parenteral amino acids during the acute phase were found to be harmful, whereby a maximal risk of harm was reached with a median daily dose of 1.15 g/kg for children <10 kg, 0.83 g/kg for children between 10–20 kg, and 0.75 g/kg for children >20 kg. Therefore, the current guidelines suggest to withhold amino acids via PN during the first week of illness (45).
After the acute phase muscle wasting often continues due to immobilization and undernourishment. Therefore, the ESPGHAN/ESPEN/ESPR/CPNN guidelines advise from day 8 onwards to provide a minimum amino acid intake of 1.0 mg/kg/min in stable term infants and 0.7 mg/kg/min in children from 1 month to 18 years to avoid a negative nitrogen balance while the maximum amino acid intake should not exceed 2.1 mg/kg/min in neonates, 1.7 mg/kg/min in infants and children up to 3 years and 1.4 mg/kg/min in older children (45).
Specific amino acids
Amino acids are classified into essential (cannot be synthesized from other elements), semi-essential and non-essential (can be synthesize from other elements). There is little evidence regarding specific amino acids administration during critical illness. Moreover, the available evidence focusses primarily on (pre)term neonates. Although, trials in adults providing glutamine, a semi-essential amino acid, as a single nutrient or in combination with other nutritional supplements did find a reduction in sepsis and mortality (46) and was found safe in 19 infants after surgical interventions (47), there seems to be no evidence for glutamine in PN in infants and young children as this failed to show a beneficial effect on outcome and is currently not advised in PN in children up to 2 years (48-50). The semi-essential amino acid arginine has, among others, a role the endogenous nitric oxide synthesis. A small study in critically ill septic children aged 6–16 years found arginine to increase arginine oxidation for the production of nitric oxide without an effect on arginine synthesis (51). Nonetheless, due to the overall lack of evidence the SCCM/ESICM guidelines advised against the use of glutamine, arginine, supplementation in children with septic shock or sepsis-associated organ dysfunction.
Carbohydrates
Carbohydrates or glucose are one of the main and preferred energy sources during health and during critical illness. Glucose levels are among others influenced by the route carbohydrates are provided and administration of glucose outside of the main feeding sources, such as medication. Plasma glucose levels are a balance between glucose utilization and exogenous glucose intake and endogenous glucose production (glycogenolysis and gluconeogenesis). During critical illness glucose metabolism is affected due to insulin resistance and β-cell dysfunction, which increases the risk of developing hyperglycaemia. Due to the restricted glucose utilisation in the acute phase lower doses are advised during this acute phase compared to the acute and stable phase. Recommended doses per phase and weight are presented for children from 28 days to 18 years in Table 2 (52). For term neonates it is recommended to start with 2.5–5 mg/kg/min gradually increasing towards 5–10 mg/kg/min. Additionally, during stable and recovery phase the concomitant provision of protein and lipids should be incorporated in the amount of glucose provision. It is important to maintain normal plasma levels of glucose as hyperglycaemia and hypoglycaemia are both associated with impaired outcomes and carbohydrate tolerance should be controlled through glycemic monitoring (<8 mmol/L in critically ill; <10 mmol/L sepsis or traumatic brain injury) (5,52).
Table 2
Phase | 28 d–10 kg | 11–30 kg | 31–45 kg | >45 kg |
---|---|---|---|---|
Acute phase (mg/kg/min) | 2–4 | 1.5–2.5 | 1–1.5 | 0.5–1 |
Stable phase (mg/kg/min) | 4–6 | 2–4 | 1.5–3 | 1–2 |
Recovery phase (mg/kg/min) | 6–10 | 3–6 | 3–4 | 2–3 |
Lipids
Parenteral lipid provision should be a fundamental part of PN in critically ill children during stable and recovery phase. Normally, lipid intake accounts for 25–50% of the non-protein caloric intake in parenterally fed patients, however, critical illness can result in acceleration of the lipid metabolism. Providing lipid emulsions is essential because this allows a high energy supply without administering high doses of carbohydrates as an iso-osmolar solution in a low volume. The supply of fatty acids, with a minimum of linoleic acid intake of 0.1 g/kg/day, is essential to prevent essential fatty acid deficiencies (53). The provided dosage of lipids should not exceed the capacity for lipid clearance and should be lowered in case of hyperlipidaemia [serum triglyceride level is >265 mg/dL (>3.0 mmol/L) in infants, >400 mg/dL (>4.5 mmol/L) in children]. It is currently advised not to exceed a lipid intake of 4 and 3 g/kg/day via PN in infants and children respectively.
Dose of parenteral micronutrients
Comparable to the macronutrients, the micronutrient needs may also differ during the course of paediatric critical illness. During the catabolic acute phase energy expenditure is altered and protein breakdown is increased. The demand for trace elements and water-soluble vitamins, which serve as coenzymes in these metabolic pathways, will rise. Simultaneously, the cell breakdown results in release of intracellular elements ensuring the availability of many elements. During anabolic phase the micronutrient need rises to allow normal of even catch-up development and patients presenting with deficiencies are more likely during the anabolic phase after a prolonged catabolic phase (54,55). Increased losses, e.g., zinc deficiency as a result of diarrhoea, potassium with vomiting, may also interfere with maintaining optimal levels.
When depletions passed the subclinical phase, it may manifest in encephalopathy, muscle weakness, neuropathy, wound healing and affect cardiac and other organ functions and as a final stage result in death (54). Critical illness and inflammation are known to have an effect on the plasma levels of micronutrients and associations with deficiencies have been made with continuous renal replacement therapy and cardiac surgery. Low micronutrient levels are reported for thiamine, riboflavin, folate, vitamin B6, vitamin B12, vitamin A, b-carotene, zinc, selenium, iron and chromium, were high or unchanged levels were found for vitamin E, vitamin B6, copper and manganese (4). The clinical interpretation of blood plasma levels can be misleading during critical illness and might not reflect true intracellular deficiencies (56). Furthermore, the actual relevance of micronutrient deficiencies or redistribution in critically ill children remains uncertain, nonetheless reported prevalence’s are high and associations have been made with adverse outcome (4,57-60).
Supplementation
Adult studies in critically ill patients confirm the association between micronutrient deficiency and stress response, however, recent RCTs and meta-analyses failed to find a causality between single or combination of supplemented micronutrients (i.e., selenium, copper, zinc, thiamine and vitamins vitamin B12, D, C & E) and clinical outcomes including mortality, length of stay and time to recover from sepsis (61-70). Several recent studies have invested in the combination of vitamin C, thiamine and hydrocortisone as a potential therapy to accelerate recovery (69,71-75). An observational study in paediatric septic patients who received vitamin C, thiamine in addition to hydrocortisone showed improved short-term outcomes compared to hydrocortisone alone (71). Though, the benefit of this supplementation therapy was not confirmed by a RCT performed in adults (69).
Because there is currently no evidence for the optimal micronutrient doses accounting for paediatric critical illness (4), the recommendations provided in the guidelines for parenteral micronutrients are based upon dietary intake recommendations for healthy children and do not account for the phase of illness, potential increased demands or altered losses (Table 3) (3,39-41,76).
Table 3
Nutrient | Term–6 m | 6–12 m | >12 m |
---|---|---|---|
Sodium | Day 1: 0–2 mmol/kg/d | 2–3 mmol/kg/d | 1–3 mmol/kg/d |
Days 2–4: 1–3 mmol/kg/d | |||
> Day 7: 2–3 mmol/kg/d | |||
Potassium | Days 1–3: 0–3 mmol/kg/d | 1–3 mmol/kg/d | 1–3 mmol/kg/d |
Days 4–7: 2–3 mmol/kg/d | |||
> Day 7: 1.5–3 mmol/kg/d | |||
Calcium | 0.8–1.5 mmol/kg/d | 0.5 mmol/kg/d | 0.25–0.4 mmol/kg/d |
Magnesium | 0.1–0.2 mmol/kg/d | 0.15 mmol/kg/d | 0.1 mmol/kg/d |
Phosphate | 0.7–1.3 mmol/kg/d | 0.5 mmol/kg/d | 0.2–0.7 mmol/kg/d |
Chloride | Day 1: 0–3 mmol/kg/d | 2–4 mmol/kg/d | 2–4 mmol/kg/d |
Day 2–4: 2–5 mmol/kg/d | |||
> Day 7: 2–3 mmol/kg/d | |||
Iron | Not recommended in short-term PN | Not recommended in short-term PN | Not recommended in short-term PN |
Zinc | 250 µg/kg/d (term—3 months) | 100 µg/kg/d (max. 5 mg/d) | 50 µg/kg/d (max. 5 mg/d) |
100 µg/kg/d (3–6 months) | |||
Copper | 20 µg/kg/d (max. 0.5 mg/d) | 20 µg/kg/d (max. 0.5 mg/d) | 20 µg/kg/d (max. 0.5 mg/d) |
Iodine | At least 1 µg/kg/d | At least 1 µg/kg/d | At least 1 µg/kg/d |
Selenium | 2–3 µg/kg/d (max. 100 µg/kg/d) | 2–3 µg/kg/d (max. 100 µg/d) | 2–3 µg/kg/d (max. 100 µg/d) |
Manganese | Max. 1 µg/kg/d | Max. 1 µg/kg/d | Max. 1 µg/kg/d |
Molybdenum | 0.25 µg/kg/d (max. 5.0 µg/d) | 0.25 µg/kg/d (max. 5.0 µg/d) | 0.25 µg/kg/d (max. 5.0 µg/d) |
Chromium | Not recommended in PN | Not recommended in PN | Not recommended in PN |
Vitamin A | 150–300 µg/kg/d | 150–300 µg/kg/d | 150 µg/d |
Vitamin D | 400 IU/d (or 40–150 IU/kg/d) | 40–150 IU/kg/d | 400–600 IU/d |
Vitamin E | 2.8–3.5 IU/kg/d | 2.8–3.5 IU/kg/d | 11 IU/d |
Vitamin K | 10 µg/kg/d | 10 µg/kg/d | 200 µg/d |
Vitamin C | 15–25 mg/kg/d | 15–25 mg/kg/d | 80 mg/d |
Thiamine | 0.35–0.5 mg/kg/d | 0.35–0.5 mg/kg/d | 1.2 mg/d |
Riboflavin | 0.15–0.2 mg/kg/d | 0.15–0.2 mg/kg/d | 1.4 mg/d |
Pyridoxine | 0.15–0.2 mg/kg/d | 0.15–0.2 mg/kg/d | 1.0 mg/d |
Niacin | 4–6.8 mg/kg/d | 4–6.8 mg/kg/d | 17 mg/d |
Vitamin B12 | 0.3 µg/kg/d | 0.3 µg/kg/d | 1 µg/d |
Pantothenic acid | 2.5 mg/kg/d | 2.5 mg/kg/d | 5 mg/d |
Biotin | 5–8 µg/kg/d | 5–8 µg/kg/d | 20 µg/d |
Folic acid | 56 µg/kg/d | 56 µg/kg/d | 140 µg/d |
Some comments can be made for specific micronutrients:
Sodium
Critically ill children are at risk to develop hyponatremia. A meta-analysis showed that isotonic maintenance fluids with sodium concentrations similar to blood plasma reduce the risk of developing hyponatraemia when compared with hypotonic intravenous fluids (77). The evidence suggests to use isotonic fluids for at least the first 24 hours of critical illness or post-operative care, while using the Holliday and Segar formula to calculate the amount of maintenance fluid required (76,78,79). In patients with excessive sodium losses sodium chloride solutions can be switched to sodium lactate or sodium acetate to decrease the chloride intake and thereby the risk of metabolic acidosis associated hyperchloraemia (76).
Iron
Due to the risk of overload via PN iron is preferably provided enterally and in children receiving short-term PN (<3 weeks) iron supplementation is not recommended (40).
Calcium, phosphorus, magnesium, potassium and vitamin B1 (thiamine)
Adequate threshold of calcium, phosphorus and magnesium are required for normal growth and bone mineralization. The risk of developing hypophosphatemia, hypomagnesemia, hypocalcaemia, and hypokalaemia is associated with the provision of nutrients. Especially high nutrient incline after a period of malnutrition placed critically ill children at risk of developing these depletions, commonly referred to as the refeeding syndrome. This syndrome is further characterized by hyperglycaemia and fluid retention causing oedema and can be managed by parenteral trace mineral supplementation and/or caloric feeding restriction (80). Vitamin B1 serve as a co-factor in the substrate oxidation and depletions are known to affect the neuro and cardiovascular system causing diseased as Beriberi, Wernicke’s and Korsakoff syndrome. During critical illness depletions in this micronutrient may occur after introduction of feeding after a period of malnutrition (81).
Zinc
Zinc serves as a cofactor for over 300 body enzymes including DNA synthesis and RNA transcription and deficiency is characterized by impaired immune function, glucose homeostasis wound healing and growth retardation. Zinc supplementation during critical illness is the only element investigated in critical ill children with two RCTs. The first trial showed in 24 critically ill children that by providing 500 µg/kg/d plasma levels could be restored to the near 50th percentile (82). While the second RCT providing whey protein, zinc, glutamine, selenium and metoclopramide versus whey protein in 298 critically ill children and found no differences on the immune status of these children. Additionally, this trial was terminated for futility before half the children were enrolled (83).
Selenium
Selenium is an essential antioxidant and serves as a cofactor for glutathione peroxidase, an enzyme that is linked to resolving oxidative tissue damage. It is also involved in iodothyronine deiodinase and thioredoxin and thereby having a role in the thyroid metabolism which is affected in the acute phase of critical illness (84). Selenium deficiency has been associated with, e.g., muscle weakness, immune disorders and carcinogenesis in adults, while selenium toxicity have been reported in association with gastrointestinal disturbance, skin lesions, liver dysfunction and paralysis (85). The only RCT performed in critically ill children is the previously described RCT which included selenium as one of the added nutrients which showed no favourable outcomes of supplementation of selenium together with whey protein, zinc, glutamine and metoclopramide (83). Systematic reviews in preterm neonates and adults showed that supplementation of selenium resulted in decreased mortality and duration of ICU stay, however supplemented amounts and methods varied substitutional and no dose recommendations were extracted (66,86).
Vitamin B12, vitamin C and vitamin D
The anti-inflammatory vitamin B12 supports macronutrient metabolism and DNA synthesis in health and deficiencies may results in anaemia and neurodegenerative demyelination. The absorption of this vitamin can be affected by gastrointestinal surgery, feeding via post-pyloric tube and using proton pump inhibitors, all common in the PICU (87). Measured plasma levels are unreliable which hinders detecting deficiencies and clinical trials regarding optimal supplementation are non-existent. The isolated provision of vitamin C has been investigated and high doses up to 66 mg/kg/hour may lead to reduced duration of mechanical ventilation and vasopressor support in critically ill adults, without reporting adverse effects. However, no effect was seen of this antioxidant on mortality in a systematic review combining the 5 RCTs (88). Vitamin D has been a topic of interest for many years in critical illness due to its important role in calcium and bone homeostasis, cardiovascular system and inflammation (89). A recent systematic review including 52 studies in critically ill children found a deficiency prevalence of 55% which was indeed associated with mortality (90). Again, when the 6 available RCTs evaluating vitamin D supplementation either enteral of parenteral in critically ill adults were combined in a systematic review, no benefit regarding recovery or mortality was found.
Besides acknowledging the potential modulatory effect of micronutrients on the acute stress response, the risk of intoxication caused by over supplementing should not be dismissed. It is an uncommon reported phenomenon during critical illness, nonetheless, safe upper intake levels most be verified to find the balance between both deficiency and toxicity (91). The limited available paediatric research restrains the guidance for lower and upper levels in the different phases of illness, therefore it might be a practical solution to aim for future research on the micronutrients who require more routine measurements in instable patients. Currently, daily or weekly laboratory measurements are advised for electrolytes (sodium, potassium, chloride, calcium, phosphorus and magnesium), trace minerals (iron, selenium, zinc and copper) and vitamin B12 (92).
Long-term consequences of PN
Children requiring long-term PN
Children requiring long-term PN are shorter and have an affected body composition and a higher fat mass compared to healthy subjects (93). Therefore, the success of PN support should be measured by body composition measurements which includes knowledge on lean body mass and fat mass and accompanied with muscle mass function and functional status (94,95). Furthermore. IFALD, cholestasis, metabolic syndrome and catheter-related bloodstream infections are commonly described long-term consequences of PN therapy in children requiring PN due to short bowel syndrome or low birth-weight infants (93,96-98). The pathogenesis is multifactorial, and association have been made with imbalances in amino acids composition, duration of PN and providing PN continuous (non-cyclical) (99).
In addition, the occurrence of cholestasis or IFALD is highly associated with intravenous lipid emulsions (ILEs) composition. Although there is no evidence suggesting an effect of different ILEs during short-term PN use on cholestasis or bilirubin levels, during long-term PN multicomponent ILEs (with fish oil) may contribute to a decrease in bilirubin levels and cholestasis (100). Furthermore, composite ILEs are found to be superior to pure soybean ILEs as they have less inflammatory properties, are immune modulating, have higher antioxidant content and prevent against cholestasis and IFALD (101,102), however, no study has assessed the pro- and anti-inflammatory effects of these different ILEs in critically ill children. Therefore, for PN lasting longer than a few days, pure soybean ILEs should not be used and composite ILEs with or without fish oil are the first-choice treatment (53). Provision of pure soybean oil ILEs can be considered in short-term PN with the knowledge that this may provide a less balanced nutrition than composite ILEs. Long-term neurocognitive development of children requiring long-term PN was investigated in 13 studies. The reported prevalence for normal neurocognitive development varied substantial and ranged between 29–100%, with 80–90% of the children in mainstream schools (97). There was no evidence favouring specific timing (cyclic or continuous) or other variables related to PN such as duration for its long-term consequences on neurocognitive development.
Critically ill children
Due to the advances in medical therapy and thereby increasing PICU survivorship, it becomes more and more important to consider long-term developmental outcomes of PN. Overall, studies investigating PICU survivors find lower scores for neurocognitive functioning as compared with a healthy population or normative scores. Additionally, health-related quality of life, physical and mental health status can also be affected after PICU admission (103). Additional to the evaluation of body composition and commonly described PN complications, the effect of PN therapy on organ function and short-term and long-term consequences should be monitored when critically ill children are concerned (104).
The PEPaNIC RCT was the first interventional study to investigate long-term developmental effects of a PN intervention. Two years after admission, PICU survivors had worse outcomes on anthropometrics, health status, and neurocognitive development as compared with matched healthy control children. Furthermore, the omission of PN during the acute phase of critical illness caused no harm and even resulted in better scores for visuomotor integration, and parent-reported executive functioning, in particular inhibitory control (105). Due to the large number of young infants in this trial and the plasticity of the developing brain, a longer assessment period was warranted to investigate the effect on all long-term physical, neurocognitive, and psychosocial developmental domains. The 4-year post-randomisation follow-up study affirmed that omitting supplemental PN during the first week of critical illness caused no harm and even resulted in less parent-reported emotional and behavioural problems (103). These emotional and behavioural problems can arise from poor executive functioning, such as poor inhibitory control which was already affected at the 2-year post-PICU time point (106,107). These clinical findings were supported by differences in telomere length and DNA methylation between children who received early-PN and late-PN, which substantiates plausible molecular basis of detrimental long-term consequences of high and early provision of parenteral macronutrients (108,109). However, further research is needed to unravel the underlying mechanisms of the long-term harm caused by high and early PN.
To be able to provide optimal PN beneficial for short-term and long-term outcomes, the timing, amount, composition and concomitant provision of EN should be integrated into a comprehensive approach incorporating all these features. First, the optimal timing should be defined for the individual patient which is now based on the PEPaNIC RCT on day 7, followed by a steady stepwise incline towards energy and protein targets to avoid refeeding syndrome.
Conclusions
Enteral intake is often insufficient in critically ill children which might result in a need for PN. Understanding the course of metabolic needs during the acute stress response is essential before providing PN. Based upon the findings of the landmark PEPaNIC RCT, the current recommendations changed to withhold PN during the first week of admission while continue to provide micronutrients (2-5). Although this parenteral macronutrient restriction during the acute phase has been found beneficial for critically ill children regarding physical and neurocognitive short-term and long-term consequences, further research is required to obtain the optimal timing, dose and composition of PN during stable and recovery phase as well as the determination of the role of parenteral micronutrients. Furthermore, cyclic feeding or pharmacologic interventions allowing autophagy are controversies to overcome.
Acknowledgments
Funding: None.
Footnote
Provenance and Peer Review: This article was commissioned by the editorial office, Pediatric Medicine for the series “Nutrition in the Critically Ill Child”. The article was sent for external peer review.
Conflicts of Interest: The authors have completed the ICMJE uniform disclosure form (available at http://dx.doi.org/10.21037/pm-20-88). The series “Nutrition in the Critically Ill Child” was commissioned by the editorial office without any funding or sponsorship. Dr. SCATV served as the unpaid Guest Editor of the series, and serves as an unpaid editorial board member of Pediatric Medicine from Oct 2019 to Sep 2021. Dr. RDE reports grants from ESPEN Research Grant, outside the submitted work. Dr. SCATV reports grants from ESPEN Research Grant, grants from Sophia Research Foundation, grants from Nutricia Research BV, outside the submitted work. Dr. KFMJ reports grants from Fonds NutshOhra, grants from Erasmus Trustfonds, grants from Nutricia Research BV, grants from AGIS Zorginnovatie, during the conduct of the study; grants from ESPEN Research Grant, grants from Sophia Research Foundation, outside the submitted work.
Ethical Statement: The authors are accountable for all aspects of the work in ensuring that questions related to the accuracy or integrity of any part of the work are appropriately investigated and resolved.
Open Access Statement: This is an Open Access article distributed in accordance with the Creative Commons Attribution-NonCommercial-NoDerivs 4.0 International License (CC BY-NC-ND 4.0), which permits the non-commercial replication and distribution of the article with the strict proviso that no changes or edits are made and the original work is properly cited (including links to both the formal publication through the relevant DOI and the license). See: https://creativecommons.org/licenses/by-nc-nd/4.0/.
References
- Joosten KF, Kerklaan D, Verbruggen SC. Nutritional support and the role of the stress response in critically ill children. Curr Opin Clin Nutr Metab Care 2016;19:226-33. [Crossref] [PubMed]
- Mehta NM, Skillman HE, Irving SY, et al. Guidelines for the Provision and Assessment of Nutrition Support Therapy in the Pediatric Critically Ill Patient: Society of Critical Care Medicine and American Society for Parenteral and Enteral Nutrition. JPEN J Parenter Enteral Nutr 2017;41:706-42. [Crossref] [PubMed]
- Mihatsch WA, Braegger C, Bronsky J, et al. ESPGHAN/ESPEN/ESPR/CSPEN guidelines on pediatric parenteral nutrition. Clin Nutr 2018;37:2303-5. [Crossref] [PubMed]
- Tume LN, Valla FV, Joosten K, et al. Nutritional support for children during critical illness: European Society of Pediatric and Neonatal Intensive Care (ESPNIC) metabolism, endocrine and nutrition section position statement and clinical recommendations. Intensive Care Med 2020;46:411-25. [Crossref] [PubMed]
- Weiss SL, Peters MJ, Alhazzani W, et al. Surviving Sepsis Campaign International Guidelines for the Management of Septic Shock and Sepsis-Associated Organ Dysfunction in Children. Pediatr Crit Care Med 2020;21:e52-e106. [Crossref] [PubMed]
- de Betue CT, van Steenselen WN, Hulst JM, et al. Achieving energy goals at day 4 after admission in critically ill children; predictive for outcome? Clin Nutr 2015;34:115-22. [Crossref] [PubMed]
- Martinez EE, Bechard LJ, Mehta NM. Nutrition algorithms and bedside nutrient delivery practices in pediatric intensive care units: an international multicenter cohort study. Nutr Clin Pract 2014;29:360-7. [Crossref] [PubMed]
- Mehta NM, Bechard LJ, Cahill N, et al. Nutritional practices and their relationship to clinical outcomes in critically ill children--an international multicenter cohort study*. Crit Care Med 2012;40:2204-11. [Crossref] [PubMed]
- Mehta NM, Bechard LJ, Zurakowski D, et al. Adequate enteral protein intake is inversely associated with 60-d mortality in critically ill children: a multicenter, prospective, cohort study. Am J Clin Nutr 2015;102:199-206. [Crossref] [PubMed]
- Mehta NM, McAleer D, Hamilton S, et al. Challenges to optimal enteral nutrition in a multidisciplinary pediatric intensive care unit. JPEN J Parenter Enteral Nutr 2010;34:38-45. [Crossref] [PubMed]
- Tume LN, Eveleens RD, Verbruggen SCAT, et al. Barriers to Delivery of Enteral Nutrition in Pediatric Intensive Care: A World Survey. Pediatr Crit Care Med 2020;21:e661-e671. [Crossref] [PubMed]
- Feng S, Cheng L, Lu H, et al. Nutritional Status and Clinical Outcomes in Children with Cancer on Admission to Intensive Care Units. Nutr Cancer 2020; Epub ahead of print. [Crossref] [PubMed]
- Ventura JC, Hauschild DB, Barbosa E, et al. Undernutrition at PICU Admission Is Predictor of 60-Day Mortality and PICU Length of Stay in Critically Ill Children. J Acad Nutr Diet 2020;120:219-29. [Crossref] [PubMed]
- Lakananurak N, Tienchai K. Incidence and risk factors of parenteral nutrition-associated liver disease in hospitalized adults: A prospective cohort study. Clin Nutr ESPEN 2019;34:81-6. [Crossref] [PubMed]
- Srinivasan V, Spinella PC, Drott HR, et al. Association of timing, duration, and intensity of hyperglycemia with intensive care unit mortality in critically ill children. Pediatr Crit Care Med 2004;5:329-36. [Crossref] [PubMed]
- Burkhalter TM, Hillman CH. A narrative review of physical activity, nutrition, and obesity to cognition and scholastic performance across the human lifespan. Adv Nutr 2011;2:201S-6S. [Crossref] [PubMed]
- Anjos T, Altmae S, Emmett P, et al. Nutrition and neurodevelopment in children: focus on NUTRIMENTHE project. Eur J Nutr 2013;52:1825-42. [Crossref] [PubMed]
- Kerklaan D, Fivez T, Mehta NM, et al. Worldwide Survey of Nutritional Practices in PICUs. Pediatr Crit Care Med 2016;17:10-8. [Crossref] [PubMed]
- van Puffelen E, Jacobs A, Verdoorn CJM, et al. International survey of De-implementation of initiating parenteral nutrition early in Paediatric intensive care units. BMC Health Serv Res 2019;19:379. [Crossref] [PubMed]
- Fivez T, Kerklaan D, Mesotten D, et al. Early versus Late Parenteral Nutrition in Critically Ill Children. N Engl J Med 2016;374:1111-22. [Crossref] [PubMed]
- van Puffelen E, Hulst JM, Vanhorebeek I, et al. Effect of late versus early initiation of parenteral nutrition on weight deterioration during PICU stay: Secondary analysis of the PEPaNIC randomised controlled trial. Clin Nutr 2020;39:104-9. [Crossref] [PubMed]
- van Puffelen E, Hulst JM, Vanhorebeek I, et al. Outcomes of Delaying Parenteral Nutrition for 1 Week vs Initiation Within 24 Hours Among Undernourished Children in Pediatric Intensive Care: A Subanalysis of the PEPaNIC Randomized Clinical Trial. JAMA Netw Open 2018;1:e182668 [Crossref] [PubMed]
- van Puffelen E, Vanhorebeek I, Joosten KFM, et al. Early versus late parenteral nutrition in critically ill, term neonates: a preplanned secondary subgroup analysis of the PEPaNIC multicentre, randomised controlled trial. Lancet Child Adolesc Health 2018;2:505-15. [Crossref] [PubMed]
- Joosten KFM, Eveleens RD, Verbruggen S. Nutritional support in the recovery phase of critically ill children. Curr Opin Clin Nutr Metab Care 2019;22:152-8. [Crossref] [PubMed]
- Hermans G, Casaer MP, Clerckx B, et al. Effect of tolerating macronutrient deficit on the development of intensive-care unit acquired weakness: a subanalysis of the EPaNIC trial. Lancet Respir Med 2013;1:621-9. [Crossref] [PubMed]
- Deretic V, Saitoh T, Akira S. Autophagy in infection, inflammation and immunity. Nat Rev Immunol 2013;13:722-37. [Crossref] [PubMed]
- Derde S, Vanhorebeek I, Guiza F, et al. Early parenteral nutrition evokes a phenotype of autophagy deficiency in liver and skeletal muscle of critically ill rabbits. Endocrinology 2012;153:2267-76. [Crossref] [PubMed]
- Van Dyck L, Vanhorebeek I, Wilmer A, et al. Towards a fasting-mimicking diet for critically ill patients: the pilot randomized crossover ICU-FM-1 study. Crit Care 2020;24:249. [Crossref] [PubMed]
- Gunst J, Derese I, Aertgeerts A, et al. Insufficient autophagy contributes to mitochondrial dysfunction, organ failure, and adverse outcome in an animal model of critical illness. Crit Care Med 2013;41:182-94. [Crossref] [PubMed]
- Furtado EC, Marchini JS, Fonseca CK, et al. Cyclic parenteral nutrition does not change the intestinal microbiota in patients with short bowel syndrome. Acta Cir Bras 2013;28:26-32. [Crossref] [PubMed]
- Bae HJ, Shin SH, Kim EK, et al. Effects of cyclic parenteral nutrition on parenteral nutrition-associated cholestasis in newborns. Asia Pac J Clin Nutr 2019;28:42-8. [PubMed]
- Collier S, Crough J, Hendricks K, et al. Use of cyclic parenteral nutrition in infants less than 6 months of age. Nutr Clin Pract 1994;9:65-8. [Crossref] [PubMed]
- Jensen AR, Goldin AB, Koopmeiners JS, et al. The association of cyclic parenteral nutrition and decreased incidence of cholestatic liver disease in patients with gastroschisis. J Pediatr Surg 2009;44:183-9. [Crossref] [PubMed]
- Just B, Messing B, Darmaun D, et al. Comparison of substrate utilization by indirect calorimetry during cyclic and continuous total parenteral nutrition. Am J Clin Nutr 1990;51:107-11. [Crossref] [PubMed]
- Stout SM, Cober MP. Metabolic effects of cyclic parenteral nutrition infusion in adults and children. Nutr Clin Pract 2010;25:277-81. [Crossref] [PubMed]
- Bendorf K, Friesen CA, Roberts CC. Glucose response to discontinuation of parenteral nutrition in patients less than 3 years of age. JPEN J Parenter Enteral Nutr 1996;20:120-2. [Crossref] [PubMed]
- Lacaille F, Gupte G, Colomb V, et al. Intestinal failure-associated liver disease: a position paper of the ESPGHAN Working Group of Intestinal Failure and Intestinal Transplantation. J Pediatr Gastroenterol Nutr 2015;60:272-83. [Crossref] [PubMed]
- de Cabo R, Mattson MP. Effects of Intermittent Fasting on Health, Aging, and Disease. N Engl J Med 2019;381:2541-51. [Crossref] [PubMed]
- Bronsky J, Campoy C, Braegger C, et al. ESPGHAN/ESPEN/ESPR/CSPEN guidelines on pediatric parenteral nutrition: Vitamins. Clin Nutr 2018;37:2366-78. [Crossref] [PubMed]
- Domellöf M, Szitanyi P, Simchowitz V, et al. ESPGHAN/ESPEN/ESPR/CSPEN guidelines on pediatric parenteral nutrition: Iron and trace minerals. Clin Nutr 2018;37:2354-9. [Crossref] [PubMed]
- Mihatsch W, Fewtrell M, Goulet O, et al. ESPGHAN/ESPEN/ESPR/CSPEN guidelines on pediatric parenteral nutrition: Calcium, phosphorus and magnesium. Clin Nutr 2018;37:2360-5. [Crossref] [PubMed]
- Preiser JC, van Zanten AR, Berger MM, et al. Metabolic and nutritional support of critically ill patients: consensus and controversies. Crit Care 2015;19:35. [Crossref] [PubMed]
- Joosten K, Embleton N, Yan W, et al. ESPGHAN/ESPEN/ESPR/CSPEN guidelines on pediatric parenteral nutrition: Energy. Clin Nutr 2018;37:2309-14. [Crossref] [PubMed]
- Vanhorebeek I, Verbruggen S, Casaer MP, et al. Effect of early supplemental parenteral nutrition in the paediatric ICU: a preplanned observational study of post-randomisation treatments in the PEPaNIC trial. Lancet Respir Med 2017;5:475-83. [Crossref] [PubMed]
- van Goudoever JB, Carnielli V, Darmaun D, et al. ESPGHAN/ESPEN/ESPR/CSPEN guidelines on pediatric parenteral nutrition: Amino acids. Clin Nutr 2018;37:2315-23. [Crossref] [PubMed]
- Novak F, Heyland DK, Avenell A, et al. Glutamine supplementation in serious illness: a systematic review of the evidence. Crit Care Med 2002;30:2022-9. [Crossref] [PubMed]
- Struijs MC, Schaible T, van Elburg RM, et al. Efficacy and safety of a parenteral amino acid solution containing alanyl-glutamine versus standard solution in infants: a first-in-man randomized double-blind trial. Clin Nutr 2013;32:331-7. [Crossref] [PubMed]
- Albers MJIJ, Steyerberg EW, Hazebroek FWJ, et al. Glutamine Supplementation of Parenteral Nutrition Does Not Improve Intestinal Permeability, Nitrogen Balance, or Outcome in Newborns and Infants Undergoing Digestive-Tract Surgery: Results From a Double-Blind, Randomized, Controlled Trial. Annals of Surgery 2005;241:599-606. [Crossref] [PubMed]
- Ong EG, Eaton S, Wade AM, et al. Randomized clinical trial of glutamine-supplemented versus standard parenteral nutrition in infants with surgical gastrointestinal disease. Br J Surg 2012;99:929-38. [Crossref] [PubMed]
- Tubman TR, Thompson SW, McGuire W. Glutamine supplementation to prevent morbidity and mortality in preterm infants. Cochrane Database Syst Rev 2008;CD001457 [Crossref] [PubMed]
- Argaman Z, Young VR, Noviski N, et al. Arginine and nitric oxide metabolism in critically ill septic pediatric patients. Crit Care Med 2003;31:591-7. [Crossref] [PubMed]
- Mesotten D, Joosten K, van Kempen A, et al. ESPGHAN/ESPEN/ESPR/CSPEN guidelines on pediatric parenteral nutrition: Carbohydrates. Clin Nutr 2018;37:2337-43. [Crossref] [PubMed]
- Lapillonne A, Fidler Mis N, Goulet O, et al. ESPGHAN/ESPEN/ESPR/CSPEN guidelines on pediatric parenteral nutrition: Lipids. Clin Nutr 2018;37:2324-36. [Crossref] [PubMed]
- Shenkin A. Micronutrients in health and disease. Postgrad Med J 2006;82:559-67. [Crossref] [PubMed]
- Kay RG, Tasman-Jones C, Pybus J, et al. A syndrome of acute zinc deficiency during total parenteral alimentation in man. Ann Surg 1976;183:331-40. [Crossref] [PubMed]
- Gerasimidis K, Bronsky J, Catchpole A, et al. Assessment and Interpretation of Vitamin and Trace Element Status in Sick Children: A Position Paper from the European Society for Paediatric Gastroenterology Hepatology, and Nutrition Committee on Nutrition. J Pediatr Gastroenterol Nutr 2020;70:873-81. [Crossref] [PubMed]
- Santana e Meneses JF, Leite HP, de Carvalho WB, et al. Hypophosphatemia in critically ill children: prevalence and associated risk factors. Pediatr Crit Care Med 2009;10:234-8. [Crossref] [PubMed]
- Dao DT, Anez-Bustillos L, Cho BS, et al. Assessment of Micronutrient Status in Critically Ill Children: Challenges and Opportunities. Nutrients 2017;9:1185. [Crossref] [PubMed]
- Byrnes MC, Stangenes J. Refeeding in the ICU: an adult and pediatric problem. Curr Opin Clin Nutr Metab Care 2011;14:186-92. [Crossref] [PubMed]
- Valla FV, Bost M, Roche S, et al. Multiple Micronutrient Plasma Level Changes Are Related to Oxidative Stress Intensity in Critically Ill Children. Pediatr Crit Care Med 2018;19:e455-63. [Crossref] [PubMed]
- Heyland D, Muscedere J, Wischmeyer PE, et al. A randomized trial of glutamine and antioxidants in critically ill patients. N Engl J Med 2013;368:1489-97. Erratum in: N Engl J Med 2013 May 9;368(19):1853. Dosage error in article text. [Crossref] [PubMed]
- van Zanten AR, Sztark F, Kaisers UX, et al. High-protein enteral nutrition enriched with immune-modulating nutrients vs standard high-protein enteral nutrition and nosocomial infections in the ICU: a randomized clinical trial. Jama 2014;312:514-24. [Crossref] [PubMed]
- Moghaddam OM, Lahiji MN, Hassani V, et al. Early Administration of Selenium in Patients with Acute Traumatic Brain Injury: A Randomized Double-blinded Controlled Trial. Indian J Crit Care Med 2017;21:75-9. [Crossref] [PubMed]
- Berger MM, Eggimann P, Heyland DK, et al. Reduction of nosocomial pneumonia after major burns by trace element supplementation: aggregation of two randomised trials. Crit Care 2006;10:R153. [Crossref] [PubMed]
- Allingstrup M, Afshari A. Selenium supplementation for critically ill adults. Cochrane Database Syst Rev 2015;2015:CD003703 [PubMed]
- Zhao Y, Yang M, Mao Z, et al. The clinical outcomes of selenium supplementation on critically ill patients: A meta-analysis of randomized controlled trials. Medicine (Baltimore) 2019;98:e15473 [Crossref] [PubMed]
- Langlois PL, Szwec C, D'Aragon F, et al. Vitamin D supplementation in the critically ill: A systematic review and meta-analysis. Clin Nutr 2018;37:1238-46. [Crossref] [PubMed]
- Fowler AA 3rd, Truwit JD, Hite RD, et al. Effect of Vitamin C Infusion on Organ Failure and Biomarkers of Inflammation and Vascular Injury in Patients with Sepsis and Severe Acute Respiratory Failure: The CITRIS-ALI Randomized Clinical Trial. JAMA 2019;322:1261-70. [Crossref] [PubMed]
- Fujii T, Luethi N, Young PJ, et al. Effect of Vitamin C, Hydrocortisone, and Thiamine vs Hydrocortisone Alone on Time Alive and Free of Vasopressor Support Among Patients With Septic Shock: The VITAMINS Randomized Clinical Trial. JAMA 2020;323:423-31. [Crossref] [PubMed]
- Campillo B, Zittoun J, de Gialluly E. Prophylaxis of folate deficiency in acutely ill patients: results of a randomized clinical trial. Intensive Care Med 1988;14:640-5. [Crossref] [PubMed]
- Wald EL, Sanchez-Pinto LN, Smith CM, et al. Hydrocortisone-Ascorbic Acid-Thiamine Use Associated with Lower Mortality in Pediatric Septic Shock. Am J Respir Crit Care Med 2020;201:863-7. [Crossref] [PubMed]
- Hager DN, Hooper MH, Bernard GR, et al. The Vitamin C, Thiamine and Steroids in Sepsis (VICTAS) Protocol: a prospective, multi-center, double-blind, adaptive sample size, randomized, placebo-controlled, clinical trial. Trials 2019;20:197. [Crossref] [PubMed]
- Marik PE, Khangoora V, Rivera R, et al. Hydrocortisone, Vitamin C, and Thiamine for the Treatment of Severe Sepsis and Septic Shock: A Retrospective Before-After Study. Chest 2017;151:1229-38. [Crossref] [PubMed]
- Balakrishnan M, Gandhi H, Shah K, et al. Hydrocortisone, Vitamin C and thiamine for the treatment of sepsis and septic shock following cardiac surgery. Indian J Anaesth 2018;62:934-9. [PubMed]
- Kim WY, Jo EJ, Eom JS, et al. Combined vitamin C, hydrocortisone, and thiamine therapy for patients with severe pneumonia who were admitted to the intensive care unit: Propensity score-based analysis of a before-after cohort study. J Crit Care 2018;47:211-8. [Crossref] [PubMed]
- Jochum F, Moltu SJ, Senterre T, et al. ESPGHAN/ESPEN/ESPR/CSPEN guidelines on pediatric parenteral nutrition: Fluid and electrolytes. Clin Nutr 2018;37:2344-53. [Crossref] [PubMed]
- McNab S, Ware RS, Neville KA, et al. Isotonic versus hypotonic solutions for maintenance intravenous fluid administration in children. Cochrane Database Syst Rev 2014;CD009457 [Crossref] [PubMed]
- Holliday MA, Segar WE. The maintenance need for water in parenteral fluid therapy. Pediatrics 1957;19:823-32. [PubMed]
- Chesney CR. The maintenance need for water in parenteral fluid therapy, by Malcolm A. Holliday, MD, and William E. Segar, MD, Pediatrics, 1957;19:823-832. Pediatrics 1998;102:229-30. [PubMed]
- Doig GS, Simpson F, Heighes PT, et al. Restricted versus continued standard caloric intake during the management of refeeding syndrome in critically ill adults: a randomised, parallel-group, multicentre, single-blind controlled trial. Lancet Respir Med 2015;3:943-52. [Crossref] [PubMed]
- Collie JTB, Greaves RF, Jones OAH, et al. Vitamin B1 in critically ill patients: needs and challenges. Clin Chem Lab Med 2017;55:1652-68. [Crossref] [PubMed]
- Cvijanovich NZ, King JC, Flori HR, et al. Safety and Dose Escalation Study of Intravenous Zinc Supplementation in Pediatric Critical Illness. JPEN J Parenter Enteral Nutr 2016;40:860-8. [Crossref] [PubMed]
- Carcillo JA, Dean JM, Holubkov R, et al. The randomized comparative pediatric critical illness stress-induced immune suppression (CRISIS) prevention trial. Pediatr Crit Care Med 2012;13:165-73. [Crossref] [PubMed]
- Jacobs A, Derese I, Vander Perre S, et al. Non-Thyroidal Illness Syndrome in Critically Ill Children: Prognostic Value and Impact of Nutritional Management. Thyroid 2019;29:480-92. [Crossref] [PubMed]
- National Research Council. Selenium in Nutrition, Revised Edition. Washington, DC: The National Academies Press, 1983. doi: https://doi.org/
10.17226/40 . - Darlow BA, Austin NC. Selenium supplementation to prevent short-term morbidity in preterm neonates. Cochrane Database Syst Rev 2003;CD003312 [Crossref] [PubMed]
- Green R, Allen LH, Bjørke-Monsen AL, et al. Vitamin B(12) deficiency. Nat Rev Dis Primers 2017;3:17040. [Crossref] [PubMed]
- Zhang M, Jativa DF. Vitamin C supplementation in the critically ill: A systematic review and meta-analysis. SAGE Open Med 2018;6:2050312118807615 [Crossref] [PubMed]
- Van den Berghe G, Van Roosbroeck D, Vanhove P, et al. Bone turnover in prolonged critical illness: effect of vitamin D. J Clin Endocrinol Metab 2003;88:4623-32. [Crossref] [PubMed]
- Razavi Khorasani N, Moazzami B, Zahedi Tajrishi F, et al. The Association Between Low Levels of Vitamin D and Clinical Outcomes in Critically-Ill Children: A Systematic Review and Meta-Analysis. Fetal Pediatr Pathol 2019; Epub ahead of print. [Crossref] [PubMed]
- Renwick AG. Toxicology of micronutrients: adverse effects and uncertainty. J Nutr 2006;136:493S-501S. [Crossref] [PubMed]
- Puntis J, Hojsak I, Ksiazyk J, et al. ESPGHAN/ESPEN/ESPR/CSPEN guidelines on pediatric parenteral nutrition: Organisational aspects. Clin Nutr 2018;37:2392-400. [Crossref] [PubMed]
- Neelis E, Kouwenhoven S, Olieman J, et al. Body Composition Using Air Displacement Plethysmography in Children with Intestinal Failure Receiving Long-Term Home Parenteral Nutrition. JPEN J Parenter Enteral Nutr 2020;44:318-26. [Crossref] [PubMed]
- Pollack MM, Holubkov R, Funai T, et al. Pediatric intensive care outcomes: development of new morbidities during pediatric critical care. Pediatr Crit Care Med 2014;15:821-7. [Crossref] [PubMed]
- Williams S, Horrocks IA, Ouvrier RA, et al. Critical illness polyneuropathy and myopathy in pediatric intensive care: A review. Pediatr Crit Care Med 2007;8:18-22. [Crossref] [PubMed]
- Lauriti G, Zani A, Aufieri R, et al. Incidence, prevention, and treatment of parenteral nutrition-associated cholestasis and intestinal failure-associated liver disease in infants and children: a systematic review. JPEN J Parenter Enteral Nutr 2014;38:70-85. [Crossref] [PubMed]
- Pierret ACS, Wilkinson JT, Zilbauer M, et al. Clinical outcomes in pediatric intestinal failure: a meta-analysis and meta-regression. Am J Clin Nutr 2019;110:430-6. [Crossref] [PubMed]
- Tang A, Slopen N, Nelson CA, et al. Catch-up growth, metabolic, and cardiovascular risk in post-institutionalized Romanian adolescents. Pediatr Res 2018;84:842-8. [Crossref] [PubMed]
- Zemrani B, Bines JE. Monitoring of long-term parenteral nutrition in children with intestinal failure. JGH Open 2019;3:163-72. [Crossref] [PubMed]
- Hojsak I, Colomb V, Braegger C, et al. ESPGHAN Committee on Nutrition Position Paper. Intravenous Lipid Emulsions and Risk of Hepatotoxicity in Infants and Children: a Systematic Review and Meta-analysis. J Pediatr Gastroenterol Nutr 2016;62:776-92. [Crossref] [PubMed]
- Manzanares W, Langlois PL, Dhaliwal R, et al. Intravenous fish oil lipid emulsions in critically ill patients: an updated systematic review and meta-analysis. Crit Care 2015;19:167. [Crossref] [PubMed]
- Diamond IR, Grant RC, Pencharz PB, et al. Preventing the Progression of Intestinal Failure-Associated Liver Disease in Infants Using a Composite Lipid Emulsion: A Pilot Randomized Controlled Trial of SMOFlipid. JPEN J Parenter Enteral Nutr 2017;41:866-77. [Crossref] [PubMed]
- Jacobs A, Dulfer K, Eveleens RD, et al. Long-term developmental effect of withholding parenteral nutrition in paediatric intensive care units: a 4-year follow-up of the PEPaNIC randomised controlled trial. Lancet Child Adolesc Health 2020;4:503-14. [Crossref] [PubMed]
- Joosten K, van Puffelen E, Verbruggen S. Optimal nutrition in the paediatric ICU. Curr Opin Clin Nutr Metab Care 2016;19:131-7. [Crossref] [PubMed]
- Verstraete S, Verbruggen SC, Hordijk JA, et al. Long-term developmental effects of withholding parenteral nutrition for 1 week in the paediatric intensive care unit: a 2-year follow-up of the PEPaNIC international, randomised, controlled trial. Lancet Respir Med 2019;7:141-53. [Crossref] [PubMed]
- Bornstein MH, Hahn CS, Suwalsky JT. Developmental Pathways among Adaptive Functioning and Externalizing and Internalizing Behavioral Problems: Cascades from Childhood into Adolescence. Appl Dev Sci 2013;17:76-87. [Crossref] [PubMed]
- Manning JC, Pinto NP, Rennick JE, et al. Conceptualizing Post Intensive Care Syndrome in Children-The PICS-p Framework. Pediatr Crit Care Med 2018;19:298-300. [Crossref] [PubMed]
- Güiza F, Vanhorebeek I, Verstraete S, et al. Effect of early parenteral nutrition during paediatric critical illness on DNA methylation as a potential mediator of impaired neurocognitive development: a pre-planned secondary analysis of the PEPaNIC international randomised controlled trial. Lancet Respir Med 2020;8:288-303. [Crossref] [PubMed]
- Verstraete S, Vanhorebeek I, van Puffelen E, et al. Leukocyte telomere length in paediatric critical illness: effect of early parenteral nutrition. Crit Care 2018;22:38. [Crossref] [PubMed]
Cite this article as: Eveleens RD, Verbruggen SCAT, Joosten KFM. The role of parenteral nutrition in paediatric critical care, and its consequences on recovery. Pediatr Med 2020;3:24.