Gastrointestinal function in critical illness—a complex interplay between the nervous and enteroendocrine systems
Introduction
Gastrointestinal (GI) dysfunction affects between 40% and 80% of critically ill children (1-3). Physiologically, GI function includes complex and coordinated interactions between the neurologic and endocrine systems resulting in digestion, absorption, and peristalsis. In critical illness, both, digestion, secondary to dysmotility, and absorption, secondary to epithelial barrier disruption, can be affected (2,4). GI dysfunction results in enteral nutrition (EN) intolerance which leads to an inability to achieve EN targets and has been associated with greater length of stay in the intensive care unit (ICU), acquired infections, and mortality (2,4,5). In this review we present an overview of GI physiology and the pathophysiology of its dysfunction during critical illness, with particular emphasis on the diagnosis and treatment of GI dysmotility in critically ill patients.
Physiology
GI motility is the result of a complex interplay between the nervous system (extrinsic and intrinsic) and the endocrine system both of which are differently modulated by feeding versus fasting states (6-10).
The extrinsic nervous system is present throughout the GI tract and includes spinal afferents and the autonomic nervous system (ANS) both parasympathetic and sympathetic innervation. The parasympathetic innervation is provided by the vagus nerve from the esophagus to the proximal large intestine, and the pelvic nerve for the remaining distal GI tract. Afferent innervation of the ANS provides signals from mechano- and chemo-receptors in the GI tract and the efferent nerves act on smooth muscle and secretory and endocrine cells. The enteric nervous system, the intrinsic nervous system of the GI tract, can also integrate motor and sensory input and act on motor, secretory and endocrine functions of the GI tract but independently of the central nervous system. Cells of the enteric nervous system are in the myenteric and submucosal plexi, where they act on motor and secretory functions, respectively, and can interact with components of the ANS. The interplay among these neural networks is demonstrated most prominently in patients with vagotomies who develop gastroparesis despite an intact enteric nervous system, and patients with Hirschsprung’s disease, who have ineffective colonic motility despite an intact ANS. In addition to the extrinsic and intrinsic nervous systems, GI motility is regulated by the interstitial cells of Cajal (ICC) and the enteroendocrine system (7,11). The ICCs, primarily present in the submucosal layers of the GI tract, serve as pacemakers generating constant slow waves that can be transformed into complete depolarizations and GI contractions with secondary stimuli (11). The enteroendocrine system is composed of different types of enterochromaffin cells (ECCs) which account for <1% of the cells of the epithelial barrier and are selectively distributed throughout the whole GI tract. Enterochromaffin cells can sense nutrients either in the apical or basolateral surface of the epithelial mucosa and release hormones or hormone-like substances that regulate GI function including motility and secretory functions (7). Figure 1 depicts the distribution of these neurologic and enteroendocrine components in the layers of the GI tract.
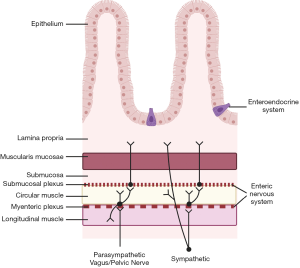
Fasting (Interdigestive) state
Under fasting conditions, or the interdigestive period, the GI tract goes through a cycle of phasic contractions known as the migrating motor complex (MMC). The MMC serves a housekeeping function whereby residual luminal content is propelled forward to clear the GI tract. The MMC is primarily initiated in the distal stomach and small intestine and is composed of three phases, a quiescent phase (45–60 minutes), a phase of irregular contractions (35–50 minutes) and a short phase of regular contractions (5–15 minutes) (6). The MMC is controlled by parasympathetic input, GI hormones, specifically motilin, ICCs, and the enteric nervous system.
Feeding state
Upon ingestion of a meal, neural and GI hormone signaling significantly change to facilitate digestion and absorption of nutrients. The MMC ceases and stretch mechanoreceptors in the proximal stomach trigger “receptive relaxation” via vago-vagal reflexes accommodating up to 1.5 L of volume in adults (6). Simultaneously, the distal stomach undergoes cycles of contraction and relaxation to break down a solid meal (6). Exposure of the small intestine to macronutrients and an osmolar load activates feedback loops within the ANS and enteroendocrine signaling, that increase the contraction and relaxation cycles and control pyloric relaxation. Pressure gradients between the antrum and the duodenum allow for controlled periodic transfer of digested food bolus into the duodenum for nutrient absorption. Parasympathetic and enteroendocrine signaling also slows down gastric emptying and the transfer of chyme from the stomach into the small intestine to ensure complete absorption of nutrients in the small intestine. GI hormones serve as a regulating mechanism for multiple phases of digestion and are released or suppressed, depending on their function, in the presence of luminal macronutrients or after absorption in different segments of the GI tract (7). The secretion of some GI hormones is also either triggered or regulated by parasympathetic input (7). Table 1 summarizes the site of and trigger for secretion or suppression of GI hormones as well as described effects on GI motility. Gastric emptying of a standard meal will take 2–3 hours, however, differences in the digestive patterns are present when comparing solid versus liquid meals and meals with different osmolar loads (6).
Table 1
Hormone | Site of secretion | Digestion phase & stimulus for secretion | Effect on gastrointestinal motility |
---|---|---|---|
Somatostatin (SST) | Whole gastrointestinal (GI) tract | Interdigestive / Feeding | Delays stomach emptying and prolongs migrating motor complex (MMC) resulting in whole GI motility slowing |
Nutrients, pH change, other hormones (GIP, GLP-1, CCK), neuropeptides (VIP, Ach) | |||
Ghrelin | Primarily stomach | Interdigestive | Stimulates stomach emptying & promotes Phase III of the MMC |
Secretion is suppressed by glucose or fat ingestion and alpha-adrenergic input/ Secretion increased by muscarinic and beta-adrenergic input | |||
Motilin | Small intestine | Interdigestive | Stimulates stomach emptying and promotes Phase III of the MMC |
Secretion suppressed by vagal input during a meal, carbohydrates, acidification of duodenal content | |||
Cholecystokinin (CCK) | Small intestine, central and peripheral nervous system | Feeding | Delays stomach emptying & inhibits antral motor activity |
Secretion stimulated the greatest by fat followed by protein, and minimally carbohydrate | Increases pyloric tone | ||
Increases small intestine transit | |||
Secretin | Small intestine | Feeding | Delays stomach emptying |
Fat and acid content in the small intestine | Increases pyloric tone | ||
Slows intestinal motility | |||
Glucagon-like peptide-1 (GLP-1) & GLP-2 | Distal small intestine and large intestine | Feeding | GLP-1 delays stomach emptying and with PYY contributes to the ‘ileal break’ |
Secretion stimulated by all macronutrients and bile acids | GLP-2 is co-secreted with GLP-1 and has a role in epithelial recovery | ||
Peptide-YY (PYY) | Distal small intestine and large intestine | Feeding | Delays stomach emptying and with GLP-1 contributes to the ‘ileal break’ |
Co-localized and secreted with GLP-1. Secretion stimulated by all macronutrients, bile acids, CCK, VIP, GLP-1 and vagus nerve. | Slows intestinal motility | ||
Amylin | Pancreas | Glucose | Delays stomach emptying |
Large Intestine Motility
The large intestine reabsorbs electrolytes and water, and allows for controlled waste of unabsorbed luminal content (12). This process, unlike that of stomach and small intestinal digestion, is prolonged and transit of luminal contents from cecum to rectum can take over 48 hours in healthy patients. Motility in the large intestine is also regulated by the enteric nervous system, ANS and GI hormone signaling. An important role of the large intestine in GI function is the secondary metabolism of nutrients by commensal bacteria. Significant advances have identified how bacterially derived metabolites contribute to GI, immune and neurologic health (13-15).
Pathophysiology
GI motility can be greatly altered in the setting of critical illness secondary to the effect of inflammation and medications on the neurologic and enteroendocrine systems. The majority of studies have demonstrated that in critically ill patients there is a slowing of gastric emptying, a loss of coordination between the antrum and the duodenum, and a slowing of colonic motility (2,3,16,17). Figure 2 presents a schematic of the multiple alterations of the extrinsic and intrinsic nervous systems and the enteroendocrine system that result in GI dysmotility during critical illness.
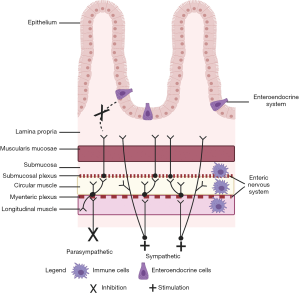
In critical illness, the sympathetic arm of the ANS is primarily activated, resulting in a suppression of parasympathetic input (18). Furthermore, medications administered in critical care have an anticholinergic effect which can further suppress the parasympathetic system (19). Inhibition of the parasympathetic nervous system results in an overall slowing down of GI functions as has been noted in patients with vagotomies and in animal models (20-22). The parasympathetic nervous system, the vagus nerve specifically, has been shown to modulate GI hormones levels. For example, experimental models have shown that vagotomy or cholinergic inhibition results in reduced ghrelin and peptide-YY (PYY) secretion (23,24). Abnormal GI hormone levels have been reported in critical illness independent of the effect of the ANS on their secretion. Table 2 summarizes adult and pediatric studies of critically ill patients where GI hormone levels were examined and correlated with GI motility or EN tolerance as a proxy measure. The most commonly studied GI hormones in critical illness are cholecystokinin (CCK), PYY, and ghrelin (26,28-32). Results vary among studies and this may be secondary to differences in study design such as sampling of hormone levels during fasting versus fed states, assays utilized for measuring hormone levels or the definition applied for GI dysmotility.
Table 2
Authors (Ref) | Study population | Clinical methods | Hormone studied | Outcome |
---|---|---|---|---|
Crona et al. (25) * | 20 adult patients with feed intolerance; 10 without | GE by AAT; Feed tolerance by GRVs | Ghrelin, CCK, motilin | Ghrelin levels were greater in feed intolerant patients; Feed intolerance correlated with delayed GE |
Nguyen et al. (26) * | 39 adult patients | GE by CO13 breath test | CCK, PYY | CCK and PYY levels greater in patients with delayed GE while fasting and feeding |
Summers et al. (27) * | 26 adult patients; 23 controls | GE by CO13 breath test; Feed tolerance by GRV and emesis | Amylin, GLP-1, glucose | GLP-1 levels were greater in feed intolerant patients |
Nguyen et al. (28) * | 19 adult patients; 24 controls | Feed intolerance by GRV | CCK, PYY | PYY and CCK levels were greater in feed intolerant patients during fasting and feeding |
Santacruz et al. (29) * | 30 adult patients; 10 controls | Feed intolerance by GRV | PYY, ghrelin | Ghrelin levels were lower in critically ill than in controls; No difference by feed intolerance |
Martinez et al. (30) ** | 14 pediatric patients | GE by AAT; Feed intolerance by EN delivery | CCK, GLP-1, PYY, GIP, glucagon, ghrelin, amylin | Greater levels GLP-1, glucagon and amylin correlated with feed intolerance; Lower PYY and ghrelin levels correlated with delayed gastric emptying |
Mayer et al. (3) ** | 23 pediatric patients | GE by AAT; Feed tolerance by GRV | Amylin | Amylin levels were greater in feed intolerant patients; Feed intolerance correlated with delayed GE |
Shanahan et al. (31) ** | 64 preterm infants <30 weeks GA | Feed intolerance by time to reach full enteral nutrition | Amylin, GIP, GLP1 GLP-2, Ghrelin, Insulin, Leptin, PYY | PYY and GIP levels were lower in feed intolerant patients |
Sharman-Koendjbiharie et al. (32) ** | 8 preterm infants with NEC and ileostomy; 11 controls | Feed intolerance by volume of feed provided | Gastrin, CCK, PYY | CCK levels were greater and PYY levels were lower when feeding volume was lower |
Hanekamp et al. (33) ** | 12 neonates on ECMO | Change between PN and EN | CCK, PYY, Gastrin | Gastrin, CCK, PYY levels were greater when EN was initiated |
Kairamkonda et al. (34) ** | 70 Preterm neonates | Feed intolerance by GRV and time to goal EN | Amylin | Amylin levels were greater in feed intolerant patients |
*, indicates adult studies; **, indicates pediatric studies. GE, gastric emptying; AAT, acetaminophen absorption test; GRV, gastric residual volume; CO13, 13-C octanoic test; PN, parenteral nutrition; EN, enteral nutrition; NEC, necrotizing enterocolitis; ECMO, extracorporeal membrane oxygenation; CCK, cholecystokinin; GLP-1, glucagon like peptide 1; GLP-2, glucagon like peptide 2; PYY, peptide-YY; GIP, total gastric inhibitory polypeptide.
The enteric nervous system can locally control smooth muscle action and interacts with the ANS to regulate motility. Enteric neurons interact with innate immune cells of the GI tract and therefore, under conditions of inflammation the activation of innate immune cells and their signaling on the enteric nervous system can result in changes in GI motility (14,35). In animal models, activated pro-inflammatory macrophages and their interactions with the enteric nervous system have been implicated in the development of post-operative ileus (36). A contributing factor to the activation of the innate immune cell population of the GI tract is the increase in intestinal trafficking of microbial products across the epithelial barrier (15). Epithelial barrier disruption and increased intestinal trafficking have been reported in critical illness, and therefore secondary GI tract inflammation via this pathway may also affect the enteric nervous system and motility (1,37). Similarly, the microbiome and associated metabolites modulate the innate immune system and secondarily regulate the enteric nervous system and its function (14). Dysbiosis has been described in critical care and therefore, regulation of the enteric nervous system and secondary GI dysmotility in this context is also possible (38).
Diagnosis
Although many clinically-approved methodologies exist to examine GI motility, few are feasible in critically ill patients. Esophageal, antro-duodenal and colonic manometry all measure pressure changes associated with regional motility, whereas the ingestible wireless motility capsules can measure whole GI transit, and Sitz markers distal GI transit (39). Studies examining GI motility in critically ill patients have focused on measuring gastric emptying. Scintigraphy, the clinically used and gold-standard test, has been employed with the use of a portable gamma-camera in an adult ICU (40-42). However, the majority of ICUs do not have the ability to perform portable scintigraphy. The 13-C octanoic acid breath test and the acetaminophen absorption test have both been moderately to strongly correlated with scintigraphy (41-43). In both assays a known concentration of either a 13-C octanoic tagged meal or acetaminophen is administered in the stomach where neither is metabolized nor absorbed. As the stomach empties, the 13-C octanoic tagged meal or acetaminophen are then transferred into the small intestine where they are absorbed and metabolized in the liver. 13-C octanoic levels are measured in exhaled breath, whereas acetaminophen levels are measured in the blood, and the concentration of these markers over time reflects gastric emptying. These assays are non-invasive and can be nearly universally applied, except in patients with liver dysfunction and/or with severe malabsorptive disorders, either of which may alter the metabolism and absorption of each agent, respectively. Ultrasound (US) has become a common diagnostic adjunct in the ICU and may serve as a non-invasive tool to examine gastric emptying in critically ill patients. More than one US approach has been used to measure gastric emptying, including measuring a change in circumference of the stomach or the width of the antrum over time after a meal or assessing the presence or absence of contents in the stomach (44-47). The majority of studies have been completed in adult or neonatal critically ill patients, therefore additional studies to examine the use of US in the pediatric ICU are needed.
In the absence of these diagnostic methods the most common proxy measures for GI dysmotility are bedside clinical assessments for EN intolerance (5,48). In pediatric critical care studies, GI symptoms such as diarrhea, emesis, abdominal distension and gastric residual volume (GRV), are the most common bedside proxy markers for EN intolerance and GI dysfunction (5). In addition, some studies utilize discontinuation of EN as a marker for GI dysfunction. Of these markers, GRV and EN delivery have been compared with a measure of gastric emptying, the acetaminophen absorption test. One study reported no difference in the median GRV between patients with and without delayed gastric emptying but identified a correlation between gastric emptying and EN delivery (2). Another study reported that patients with GRVs greater than 125% of the instilled bolus of formula had delayed gastric emptying (3). Furthermore, a study comparing EN advancement between two pediatric ICUs with differing practices on measuring GRV found no difference in the frequency of aspiration events and greater EN delivery when GRV was not measured (49).
Treatment
Despite advances in the understanding of the physiology of GI dysmotility in critical illness, the available treatment strategies are limited. Prokinetic agents have been reported to be used in 79% of critically ill children with EN intolerance (50). The most common agents used are erythromycin, metoclopramide and domperidone (51). These agents act on one or multiple of the pathways responsible for GI dysmotility. Specifically, erythromycin is a motilin agonist, and metoclopramide and domperidone are dopamine antagonists. A meta-analysis of randomized-controlled trials including critically ill adults concluded that prokinetic agents reduced EN intolerance and the risk for high volume GRVs (52). There are insufficient pediatric ICU data on the efficacy of these medications, despite their wide use. In addition, the efficacy of these agents is short-lived as they are prone to tachyphylaxis and they carry the risk of moderate to severe side effects including prolonged QTc and tardive dyskinesia for metoclopramide, and antibiotic resistance for erythromycin. Novel prokinetic agents have been developed based on research advances on the role of GI hormones on motility. These have included ghrelin agonists, motilin agonists and serotonin agonists with reduced side effect profiles. Early clinical trials on such alternative drugs have been promising with some showing similar efficacy in improving EN tolerance and delivery compared to current agents (53-57).
An alternative strategy to administration of prokinetic agents is post-pyloric feeding to bypass EN intolerance due to delayed gastric emptying. Post-pyloric feeding has been reported in 36% of pediatric critical care patients (50). A single-center experience has shown that post-pyloric tube placement can be feasible at the bedside and safe (58). However, some argue that post-pyloric feeding, and continuous over bolus feeding, contribute to GI dysmotility in the ICU. Post-pyloric and continuous feeds both deviate from normal GI physiology and therefore may alter the complex neuro-hormone signaling that promotes normal GI motility. Compared to healthy subjects, critically ill adults on post-pyloric nutrition had slowed gastric emptying and greater antro-duodenal discoordination (17). In a cross-over study of healthy adults, continuous as compared to bolus EN was associated with lower peak of serum levels of PYY and a lesser decrease in ghrelin levels from the fasting state (59). Gastric emptying, based on gastric volume measured by magnetic resonance imaging (MRI), had a significant greater change over time in the bolus than the continuously fed cohort (59). In critically ill patients, no difference in the frequency of GI symptoms suggestive of dysfunction and EN intolerance, such as GRV or emesis, has been reported between bolus and continuously fed patients, though one recent pediatric study reported greater caloric delivery (60,61). More research is needed to understand the impact of feeding methods on abnormal GI function and its clinical relevance.
Conclusions
GI dysfunction is prevalent in critical illness and is secondary to an alteration in the complex coordination of the nervous and endocrine systems in the setting of inflammation, medications and non-physiologic alternatives to EN. Research advancements on the interplay between these systems will continue to promote the development of innovative approaches to manage GI dysfunction and prevent associated adverse outcomes.
Acknowledgments
Funding: None.
Footnote
Provenance and Peer Review: This article was commissioned by the Guest Editors (Lyvonne Tume, Frederic Valla and Sascha Verbruggen) for the series “Nutrition in the Critically Ill Child” published in Pediatric Medicine. The article was sent for external peer review organized by the Guest Editors and the editorial office.
Conflicts of Interest: The authors have completed the ICMJE uniform disclosure form (available at http://dx.doi.org/10.21037/pm-20-74). The series “Nutrition in the Critically Ill Child” was commissioned by the editorial office without any funding or sponsorship. Dr. Fasano reports the following relationships or activities: Alba Therapeutics - Alternative treatments to gluten free diet (GFD) for celiac patients | Stock holder; Mead Johnson Nutrition - Role of nutrition on inflammation | Speaking Agreement; Viome - Role of microbiome in CID | Scientific Advisory Board. The authors have no other conflicts of interest to declare.
Ethical Statement: The authors are accountable for all aspects of the work in ensuring that questions related to the accuracy or integrity of any part of the work are appropriately investigated and resolved.
Open Access Statement: This is an Open Access article distributed in accordance with the Creative Commons Attribution-NonCommercial-NoDerivs 4.0 International License (CC BY-NC-ND 4.0), which permits the non-commercial replication and distribution of the article with the strict proviso that no changes or edits are made and the original work is properly cited (including links to both the formal publication through the relevant DOI and the license). See: https://creativecommons.org/licenses/by-nc-nd/4.0/.
References
- Martinez EE, Zurakowski D, Pereira L, et al. Interleukin-10 and Zonulin Are Associated With Postoperative Delayed Gastric Emptying in Critically Ill Surgical Pediatric Patients: A Prospective Pilot Study. JPEN J Parenter Enteral Nutr 2020;44:1407-16. [Crossref] [PubMed]
- Martinez EE, Pereira LM, Gura K, et al. Gastric Emptying in Critically Ill Children. JPEN J Parenter Enteral Nutr 2017;41:1100-9. [Crossref] [PubMed]
- Mayer AP, Durward A, Turner C, et al. Amylin is associated with delayed gastric emptying in critically ill children. Intensive Care Med 2002;28:336-40. [Crossref] [PubMed]
- Typpo KV, Larmonier CB, Deschenes J, et al. Clinical characteristics associated with postoperative intestinal epithelial barrier dysfunction in children with congenital heart disease. Pediatr Crit Care Med 2015;16:37-44. [Crossref] [PubMed]
- Eveleens RD, Joosten KFM, de Koning BAE, et al. Definitions, predictors and outcomes of feeding intolerance in critically ill children: A systematic review. Clin Nutr 2020;39:685-93. [Crossref] [PubMed]
- Hennig GW, Spencer NJ. Physiology of Gastric Motility Patterns. In: Said HM. editor. Physiology of the Gastrointestinal Tract. 1. 6th ed: Elsevier, 2018.
- Gribble FM, Reimann F, Roberts GP. Gastrointestinal Hormones. In: Said HM. editor. Physiology of the Gastrointestinal Tract. 1. 6th ed: Elsevier, 2018.
- Kumral D, Zfass AM. Gut Movements: A Review of the Physiology of Gastrointestinal Transit. Dig Dis Sci 2018;63:2500-6. [Crossref] [PubMed]
- Martinez EE, Douglas K, Nurko S, et al. Gastric Dysmotility in Critically Ill Children: Pathophysiology, Diagnosis, and Management. Pediatr Crit Care Med 2015;16:828-36. [Crossref] [PubMed]
- Miller L, Roland BC, Whitson M et al. Clinical and Translational Aspects of Normal and Abnormal Motility in the Esophagus, Small Intestine and Colon. In: Said HM. editor. Physiology of the Gastrointestinal Tract. 1. 6th ed: Elsevier, 2018.
- Huizinga JD. The Physiology and Pathophysiology of Intestitial Cells of Cajal- pacemaking, innervation, and stretch sensation. In: Said HM, editor. The Physiology of the Gastrointestinal Tract. 1. 6th ed: Elsevier, 2018.
- Bharucha AE, Brookes SJ. Neurophysiologic Mechanisms of Human Large Intestinal Motility. In: Said HM, editor. Physiology of the Gastrointestinal Tract. 1. 6th ed: Elsevier, 2018.
- Cani PD, Everard A, Duparc T. Gut microbiota, enteroendocrine functions and metabolism. Curr Opin Pharmacol 2013;13:935-40. [Crossref] [PubMed]
- Muller PA, Koscso B, Rajani GM, et al. Crosstalk between muscularis macrophages and enteric neurons regulates gastrointestinal motility. Cell 2014;158:300-13. [Crossref] [PubMed]
- Mortha A, Chudnovskiy A, Hashimoto D, et al. Microbiota-dependent crosstalk between macrophages and ILC3 promotes intestinal homeostasis. Science 2014;343:1249288 [Crossref] [PubMed]
- Ritz MA, Fraser R, Tam W, et al. Impacts and patterns of disturbed gastrointestinal function in critically ill patients. Am J Gastroenterol 2000;95:3044-52. [Crossref] [PubMed]
- Chapman M, Fraser R, Vozzo R, et al. Antro-pyloro-duodenal motor responses to gastric and duodenal nutrient in critically ill patients. Gut 2005;54:1384-90. [Crossref] [PubMed]
- Badke CM, Marsillio LE, Weese-Mayer DE, et al. Autonomic Nervous System Dysfunction in Pediatric Sepsis. Front Pediatr 2018;6:280. [Crossref] [PubMed]
- Madden K, Hussain K, Tasker RC. Anticholinergic Medication Burden in Pediatric Prolonged Critical Illness: A Potentially Modifiable Risk Factor for Delirium. Pediatr Crit Care Med 2018;19:917-24. [Crossref] [PubMed]
- Ott L, Young B, Phillips R, et al. Altered gastric emptying in the head-injured patient: relationship to feeding intolerance. J Neurosurg. 1991;74:738-42. [Crossref] [PubMed]
- Robinson-Papp J, Nmashie A, Pedowitz E, et al. Vagal dysfunction and small intestinal bacterial overgrowth: novel pathways to chronic inflammation in HIV. Aids 2018;32:1147-56. [Crossref] [PubMed]
- Sugawara K, Isaza J, Woodward ER, et al. The short-term effect of vagotomy of gastric motility. Arch Surg. 1969;99:1-5. [Crossref] [PubMed]
- Hosoda H, Kangawa K. The autonomic nervous system regulates gastric ghrelin secretion in rats. Regul Pept 2008;146:12-8. [Crossref] [PubMed]
- Kim HH, Park MI, Lee SH, et al. Effects of vagus nerve preservation and vagotomy on peptide YY and body weight after subtotal gastrectomy. World J Gastroenterol 2012;18:4044-50. [Crossref] [PubMed]
- Crona D, MacLaren R. Gastrointestinal hormone concentrations associated with gastric feeding in critically ill patients. JPEN J Parenter Enteral Nutr 2012;36:189-96. [Crossref] [PubMed]
- Nguyen NQ, Fraser RJ, Bryant LK, et al. The relationship between gastric emptying, plasma cholecystokinin, and peptide YY in critically ill patients. Crit Care 2007;11:R132. [Crossref] [PubMed]
- Summers MJ, Bartolomeo AE, Zaknic AV, et al. Endogenous amylin and glucagon-like peptide-1 concentrations are not associated with gastric emptying in critical illness. Acta Anaesthesiol Scand 2014;58:235-42. [Crossref] [PubMed]
- Nguyen NQ, Fraser RJ, Chapman M, et al. Fasting and nutrient-stimulated plasma peptide-YY levels are elevated in critical illness and associated with feed intolerance: an observational, controlled study. Crit Care 2006;10:R175. [Crossref] [PubMed]
- Santacruz CA, Quintairos A, Righy C, et al. Is There a Role for Enterohormones in the Gastroparesis of Critically Ill Patients? Crit Care Med 2017;45:1696-701. [Crossref] [PubMed]
- Martinez EE, Panciotti C, Pereira LM, et al. Gastrointestinal Hormone Profiles Associated With Enteral Nutrition Tolerance and Gastric Emptying in Pediatric Critical Illness: A Pilot Study. JPEN J Parenter Enteral Nutr 2020;44:472-80. [Crossref] [PubMed]
- Shanahan KH, Yu X, Miller LG, et al. Early Serum Gut Hormone Concentrations Associated With Time to Full Enteral Feedings in Preterm Infants. J Pediatr Gastroenterol Nutr 2018;67:97-102. [Crossref] [PubMed]
- Sharman-Koendjbiharie M, Piena-Spoel M, Hopman WP, et al. Gastrointestinal hormone secretion after surgery in neonates with congenital intestinal anomalies during starvation and introduction of enteral nutrition. J Pediatr Surg 2003;38:1602-6. [Crossref] [PubMed]
- Hanekamp MN, Spoel M, Sharman-Koendjbiharie M, et al. Gut hormone profiles in critically ill neonates on extracorporeal membrane oxygenation. J Pediatr Gastroenterol Nutr 2005;40:175-9. [Crossref] [PubMed]
- Kairamkonda VR, Deorukhkar A, Bruce C, et al. Amylin peptide is increased in preterm neonates with feed intolerance. Arch Dis Child Fetal Neonatal Ed 2008;93:F265-70. [Crossref] [PubMed]
- Phillips RJ, Powley TL. Macrophages associated with the intrinsic and extrinsic autonomic innervation of the rat gastrointestinal tract. Auton Neurosci 2012;169:12-27. [Crossref] [PubMed]
- Stein K, Lysson M, Schumak B, et al. Leukocyte-Derived Interleukin-10 Aggravates Postoperative Ileus. Front Immunol 2018;9:2599. [Crossref] [PubMed]
- Doig CJ, Sutherland LR, Sandham JD, et al. Increased intestinal permeability is associated with the development of multiple organ dysfunction syndrome in critically ill ICU patients. Am J Respir Crit Care Med. 1998;158:444-51. [Crossref] [PubMed]
- Rogers MB, Firek B, Shi M, et al. Disruption of the microbiota across multiple body sites in critically ill children. Microbiome 2016;4:66. [Crossref] [PubMed]
- Keller J, Bassotti G, Clarke J, et al. Expert consensus document: Advances in the diagnosis and classification of gastric and intestinal motility disorders. Nat Rev Gastroenterol Hepatol 2018;15:291-308. [Crossref] [PubMed]
- Nguyen NQ, Chapman MJ, Fraser RJ, et al. The effects of sedation on gastric emptying and intra-gastric meal distribution in critical illness. Intensive Care Med 2008;34:454-60. [Crossref] [PubMed]
- Chapman MJ, Besanko LK, Burgstad CM, et al. Gastric emptying of a liquid nutrient meal in the critically ill: relationship between scintigraphic and carbon breath test measurement. Gut 2011;60:1336-43. [Crossref] [PubMed]
- Nguyen NQ, Bryant LK, Burgstad CM, et al. Gastric emptying measurement of liquid nutrients using the (13)C-octanoate breath test in critically ill patients: a comparison with scintigraphy. Intensive Care Med 2013;39:1238-46. [Crossref] [PubMed]
- Nimmo WS, Heading RC, Wilson J, et al. Inhibition of gastric emptying and drug absorption by narcotic analgesics. Br J Clin Pharmacol. 1975;2:509-13. [Crossref] [PubMed]
- Hamada SR, Garcon P, Ronot M, et al. Ultrasound assessment of gastric volume in critically ill patients. Intensive Care Med 2014;40:965-72. [Crossref] [PubMed]
- Liu Y, Gao YK, Yao L, et al. Modified B-ultrasound method for measurement of antral section only to assess gastric function and guide enteral nutrition in critically ill patients. World J Gastroenterol 2017;23:5229-36. [Crossref] [PubMed]
- Perrella SL, Hepworth AR, Simmer KN, et al. Repeatability of gastric volume measurements and intragastric content using ultrasound in preterm infants. J Pediatr Gastroenterol Nutr 2014;59:254-63. [Crossref] [PubMed]
- Van de Putte P, Perlas A. Ultrasound assessment of gastric content and volume. Br J Anaesth 2014;113:12-22. [Crossref] [PubMed]
- Tume LN, Valla FV. A review of feeding intolerance in critically ill children. Eur J Pediatr 2018;177:1675-83. [Crossref] [PubMed]
- Tume LN, Bickerdike A, Latten L, et al. Routine gastric residual volume measurement and energy target achievement in the PICU: a comparison study. Eur J Pediatr 2017;176:1637-44. [Crossref] [PubMed]
- Mehta NM, Bechard LJ, Zurakowski D, et al. Adequate enteral protein intake is inversely associated with 60-d mortality in critically ill children: a multicenter, prospective, cohort study. Am J Clin Nutr 2015;102:199-206. [Crossref] [PubMed]
- Martinez EE, Bechard LJ, Mehta NM. Nutrition algorithms and bedside nutrient delivery practices in pediatric intensive care units: an international multicenter cohort study. Nutr Clin Pract 2014;29:360-7. [Crossref] [PubMed]
- Lewis K, Alqahtani Z, McIntyre L, et al. The efficacy and safety of prokinetic agents in critically ill patients receiving enteral nutrition: a systematic review and meta-analysis of randomized trials. Crit Care 2016;20:259. [Crossref] [PubMed]
- Chapman MJ, Deane AM, O'Connor SL, et al. The effect of camicinal (GSK962040), a motilin agonist, on gastric emptying and glucose absorption in feed-intolerant critically ill patients: a randomized, blinded, placebo-controlled, clinical trial. Crit Care 2016;20:232. [Crossref] [PubMed]
- Deane A, Young R, Chapman M, et al. A clinical audit of the efficacy of tegaserod as a prokinetic agent in the intensive care unit. Crit Care Resusc 2008;10:71. [PubMed]
- Chapman MJ, Jones KL, Almansa C, et al. Blinded, Double-Dummy, Parallel-Group, Phase 2a Randomized Clinical Trial to Evaluate the Efficacy and Safety of a Highly Selective 5-Hydroxytryptamine Type 4 Receptor Agonist in Critically Ill Patients With Enteral Feeding Intolerance JPEN J Parenter Enteral Nutr 2020; [published online ahead of print, 2020 Jan 28]. [Crossref] [PubMed]
- Deane AM, Chapman MJ, Fraser RJ, et al. Effects of exogenous glucagon-like peptide-1 on gastric emptying and glucose absorption in the critically ill: relationship to glycemia. Crit Care Med 2010;38:1261-9. [Crossref] [PubMed]
- Heyland DK, van Zanten ARH, Grau-Carmona T, et al. A multicenter, randomized, double-blind study of ulimorelin and metoclopramide in the treatment of critically ill patients with enteral feeding intolerance: PROMOTE trial. Intensive Care Med 2019;45:647-56. [Crossref] [PubMed]
- Turner AD, Hamilton SM, Callif C, et al. Bedside Postpyloric Tube Placement and Enteral Nutrition Delivery in the Pediatric Intensive Care Unit. Nutr Clin Pract 2020;35:299-305. [Crossref] [PubMed]
- Chowdhury AH, Murray K, Hoad CL, et al. Effects of Bolus and Continuous Nasogastric Feeding on Gastric Emptying, Small Bowel Water Content, Superior Mesenteric Artery Blood Flow, and Plasma Hormone Concentrations in Healthy Adults: A Randomized Crossover Study. Ann Surg 2016;263:450-7. [Crossref] [PubMed]
- Kadamani I, Itani M, Zahran E, et al. Incidence of aspiration and gastrointestinal complications in critically ill patients using continuous versus bolus infusion of enteral nutrition: a pseudo-randomised controlled trial. Aust Crit Care 2014;27:188-93. [Crossref] [PubMed]
- Brown AM, Fisher E, Forbes ML. Bolus vs Continuous Nasogastric Feeds in Mechanically Ventilated Pediatric Patients: A Pilot Study. JPEN J Parenter Enteral Nutr 2019;43:750-8. [Crossref] [PubMed]
Cite this article as: Martinez EE, Fasano A, Mehta NM. Gastrointestinal function in critical illness—a complex interplay between the nervous and enteroendocrine systems. Pediatr Med 2020;3:23.