Infant spinal anesthesia: a narrative review
Introduction
Background
Spinal anesthesia is a neuraxial anesthetic technique that consists of a single injection of local anesthetic into the subarachnoid space. The subarachnoid space, also known as the intrathecal space, contains cerebrospinal fluid (CSF). Depositing local anesthetic in the subarachnoid space provides a dense sympathetic, sensory, and motor blockade to the abdomen, pelvis, and lower extremities. Spinal anesthesia is differentiated from caudal or epidural neuraxial anesthesia by the location of local anesthetic, level of the block, and potency among other factors (Table 1). Because the spinal anesthesia is used as a single shot, the duration is typically less than 90 minutes. Although not common in the U.S., many practices around the world routinely use adjuvants to prolong the duration of spinal anesthesia (3,4).
Table 1
Block | Caudal | Epidural | Spinal |
---|---|---|---|
Duration in infants | Up to 4 hours (1) | Up to 6 hours (can be extended by catheter) (2) | Typically, <90 minutes (2) |
Continuous catheter or single injection | Commonly single shot (catheter used in neonates) | Commonly continuous infusion (single shot used for certain indications) | Single injection |
Location | Caudal epidural space | Thoracic/lumbar epidural space (cervical epidural used mostly for pain management) | Subarachnoid space |
Efficacy | Lower | Lower | Higher |
Level | Sacral hiatus | Can be placed at thoracic, lumbar or, sacral levels and rarely at cervical levels | Although in theory can be placed anywhere, in practice is placed at lumbar or sacral level to avoid puncture of spinal cord |
Rationale and knowledge gap
The target audience of published review articles on spinal anesthesia in infants are often directed towards other anesthesiologists (5-7). In this article, we aim to highlight information relevant to other practitioners who may interact with infants receiving spinal anesthesia outside of the perioperative period (hospitalists, primary care providers, surgeons, neonatologists, etc.).
Objectives
We will review relevant history, anatomy, technique, indications, contraindications, and outcomes for infants receiving spinal anesthesia compared to a general anesthetic. We present this article in accordance with the Narrative Review reporting checklist (available at https://pm.amegroups.com/article/view/10.21037/pm-24-28/rc).
Methods
A literature search was performed utilizing MeSH, Cochrane, Google Scholar, and PubMed for articles between January 1st, 1990 and December 31st, 2022 (Table 2). Article types included randomized controlled trials, cohorts, meta-analyses, and reviews. Additional articles were found via references from articles related to the original search. There were no affiliations or conflicts of interest with the authors of the articles used. Search terms included but were not limited to “anesthesia neurodevelopment”, “anesthesia neurotoxicity”, “infant spinal anesthesia”, “neonatal spinal anesthesia”, and “pediatric spinal anesthesia”. A total of 1116 articles were identified. Of these, 168 titles and abstracts were relevant to our review. Of these articles, we identified 51 articles for use in the current review (Figure 1).
Table 2
Items | Specification |
---|---|
Date of search | October 1st, 2023–November 1st, 2023 |
Databases and other sources searched | MeSH, Cochrane, Google Scholar, and PubMed |
Search terms used | “Anesthesia neurodevelopment”, “anesthesia neurotoxicity”, “infant spinal anesthesia”, “neonatal spinal anesthesia”, and “pediatric spinal anesthesia” |
Timeframe | January 1st, 1990–December 31st, 2022 |
Inclusion and exclusion criteria | Inclusion: English language, focused on search terms |
Exclusion: neuraxial anesthesia involving adolescents and adults | |
Selection process | Literature search was conducted by the authors with inclusion based on agreement among the group |
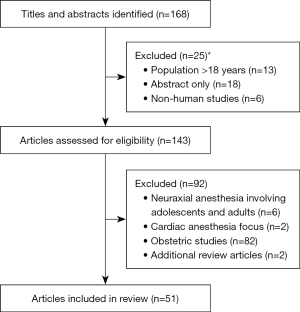
History
In 1899, Bier authored a seminal paper on spinal anesthesia entitled “Experiment regarding the cocainization of the spinal cord” (8). The use of spinal anesthesia remained a popular modality for lower extremity and abdominal surgeries until the 1940s. Improvements in the safety of general anesthetics and case reports of paraplegia related to spinal anesthesia made general anesthesia an appealing viable alternative (9). While a 1960 paper (10) showing the safety of spinal anesthesia rekindled interest in adult spinal anesthesia, it was not until the 1980s that it regained popularity in the pediatric population. In 1984, Abajian described that spinal anesthesia was an effective alternative to general anesthesia in premature infants to avoid postoperative apnea (POA) and bradycardia (11,12). While the difference in risk of POA for general and spinal anesthesia has not been shown to be significant in the late postoperative period, interest in spinal anesthesia has continued to grow due to new applications and considerations (13).
Spinal anesthesia in children has also seen renewed interest due to evidence in animal models that general anesthetic agents may cause damage to developing neuronal tissue and have potential long-term effects on neurocognitive function. In December 2016, the Food and Drug Administration (FDA) issued a statement warning that “repeated or lengthy use of general anesthetic and sedation drugs during surgeries or procedures in children younger than 3 years or in pregnant women during their third trimester may affect the development of children’s brains” (FDA 2017). While animal studies have shown potential negative effects from anesthesia on the developing brain, there has been no clear evidence showing negative effects of general anesthesia on the developing human brain. Recent studies have aimed to determine the risks of general anesthesia on the developing brain by comparing patients that receive general anesthesia to those that receive regional anesthesia. These cohort studies and clinical trials have not shown a clear benefit with one technique versus the other (14-17).
Neonatal anatomy
The spine consists of seven cervical, 12 thoracic, five lumbar, and five fused sacral vertebrae. In infants, the spinal cord terminates at the L3 vertebra and the dural sac terminates at the S3 vertebra. From the skin to the subarachnoid space, there are several layers that need to be traversed for proper placement of a spinal anesthetic: skin, subcutaneous tissue, supraspinous ligament, interspinous ligament, ligamentum flavum, epidural space, dura mater, arachnoid mater and finally subarachnoid space. In epidural and caudal anesthesia, local anesthesia is deposited into the epidural space, which is a potential space in between the ligamentum flavum and dura mater. Epidural and caudal anesthesia act by slow diffusion across the dura mater to primarily affect the spinal nerves and the spinal cord/paravertebral nerves to a lesser extent. In contrast, spinal anesthesia is deposited directly into the subarachnoid space (Figure 2), where it exerts its effects on both the spinal nerves and spinal cord. Because spinal anesthesia causes direct absorption of the local anesthetic on the spinal cord, it provides a denser block on sympathetic, sensory, and motor nerves than epidural or caudal anesthesia. As previously mentioned, the spinal cord and dural sac end at L3 and S3 respectively. In order to minimize the risk of direct injury to the spinal cord, spinal anesthesia must be performed below the level of L3, but above the level of S1 in order to ensure access to the subarachnoid space. Landmark-based techniques are most commonly used for choosing an initial insertion site as they are generally consistent across patients and indicate an intervertebral space below the L3 vertebral body. The intercristal line, also known as Tuffier’s line, is a horizontal line between the highest points of the iliac crests. It correlates roughly to the L4–5 intervertebral space in infants making it an excellent landmark and starting point for placement of the spinal needle.
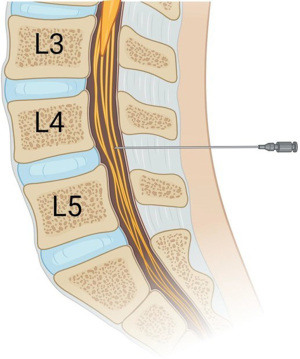
Technique
The technique of needle placement for spinal anesthesia is like that of a neonatal lumbar puncture. The baby is placed in either a sitting position, where the patient is held upright, or in a lateral position with the legs tucked and the back arched towards the provider. Ensuring that the patient is held stationary in optimal position is key to successful placement. The intervertebral space is extremely small and minor deviations from flexion, extension, or lateral rotation can easily lead to technical failure. After landmarks are palpated, the back is prepared with a cleaning solution and draped using sterile technique. Local anesthetic cream may be placed on the skin 30 minutes prior to the procedure to minimize pain (18). Caution must be used with regard to the type of local anesthesia present in the cream, the amount of cream used, the surface area of skin it is applied to, and the duration of time it is in contact with skin in order to avoid adverse events. There have been reports of methemoglobinemia because of systemic absorption of prilocaine, which is commonly used in topical anesthetic creams (19,20). After the patient is prepared in a sterile fashion and draped, the spinal needle is inserted in the intervertebral space and advanced until the subarachnoid space is reached. At this point, there will be free flow of CSF. The practitioner then injects the local anesthetic into the subarachnoid space, withdraws the needle, and lays the patient flat on his or her back to ensure the local anesthetic settles into the desired location (21). Typically, long-acting local anesthetics are used such as tetracaine, bupivacaine, or ropivacaine. If the patient remains sitting or the legs are elevated for a prolonged period of time, the local anesthesia may travel and settle in a different location than intended. This may result in an ineffective block (saddle block), or a “high spinal” in which the patient is unable to breathe due to blockade of the cervical spinal nerves which innervate the diaphragm (21). Even with long-acting local anesthetics, duration is limited; therefore, other additive medications such as epinephrine, clonidine, dexmedetomidine, and narcotics can be utilized to improve the duration of action (4). After successful spinal anesthesia, an intravenous (IV) can be placed in the lower extremity as this area is now insensate. The infants will often fall asleep during these procedures, which is theorized to be due to blockade of the somatosensory transmission through the reticulo-thalamo-cortical pathway (22). For patients that do not fall asleep, they can often be calmed by utilizing oral glucose solutions or supplemental IV or gas anesthetics during the course of the procedure. Various agents can be given preoperatively such as oral midazolam, intranasal fentanyl, or intranasal dexmedetomidine which may help prolong sedation for the procedure (23). However, the effects that these medications have on outcomes like POA is unclear.
Indications and contraindications
Types of surgeries amenable to spinal anesthesia include abdominal (2), lower extremity (21), urologic (24), and spinal defects (25). There have been reports of its use for patent ductus arteriosus closure (26). Absolute contraindications to spinal anesthesia include parent refusal, infection at needle insertion site, and increased intracranial pressure. Relative contraindications include bleeding disorders, thrombocytopenia, or systemic anticoagulation (depending on the degree of severity, class of anticoagulant, and timing from last dose). Spinal anesthesia should not be used for procedures that require controlled breathing/muscle relaxation (i.e., laparoscopic surgeries) or are routinely longer than 90 minutes in duration.
Outcomes
Safety of placement
Overall, spinal anesthesia is a safe and effective technique in infants and children (27,28). At institutions that commonly perform spinal anesthetics, the success rate is as high as 95.4% (29). While traumatic insertion may occur resulting in bleeding, this is rarely clinically significant. Cerebral spinal fluid leak into the epidural space is common in diagnostic lumbar puncture with one study showing CSF in the epidural space by ultrasound in 21 of 33 neonates within 24 hours after lumbar puncture (30). While there is no similar study for spinal anesthesia, there is one case report of a visible subcutaneous collection of CSF (31). Whereas in an older child or adult this may result in a postdural puncture headache (PDPH) due to low CSF pressure, this is not necessarily the case in pediatrics. The low incidence in children is thought to be due to low CSF pressure, elastic dura, and an inability to ambulate. Given the difficulty in diagnosing PDPH in infants, this side effect is likely underdiagnosed with the youngest reported PDPH being in a 23-month-old (32). Infection (meningitis or arachnoiditis) rarely occurs in infants with only isolated case reports present in literature (33). Of these, it is unclear whether this occurred due to the spinal anesthetic.
Hemodynamic stability
While high spinal anesthesia in adults may be associated with bradycardia and hypotension especially with blockade of the T3/4 spinal nerves, infants tolerate higher thoracic levels with minimal heart rate and blood pressure changes (34,35). This is most likely multifactorial. Neonates and infants have an underdeveloped sympathetic system and have a lower volume reservoir in the splanchnic/lower extremity vasculature as compared to adults. Therefore, when spinal anesthesia causes sympathetic blockade, there is little change in pre-load. A more recent study examining spinal anesthesia’s effect on regional cerebral oxygen saturation (rScO2) demonstrated minimal change in rScO2 based on near-infrared spectroscopy despite lower mean arterial pressure after spinal anesthesia (36). In contrast, general anesthesia results in significantly more hypotension and an increased number of interventions to treat hypotension than awake regional anesthesia techniques in infants (37,38).
Resource utilization
The ability of spinal anesthesia to obviate the need for induction, airway management, and emergence from volatile anesthetics can result in an overall decrease in time in the operating room and postoperative care unit. Kachko et al. [2009] and Ing et al. [2016] showed a reduction in operating room time for pyloromyotomies of 18.6 and 17.5 minutes, respectively (39,40). Meta-analysis of inguinal herniorrhaphy under spinal anesthesia similarly demonstrated a reduction in overall operating room time (38). In cost analysis, another study demonstrated a reduction in anesthesia cost of about 54% for infants undergoing pyloromyotomy under spinal anesthesia versus general anesthesia (34).
Neurocognitive function
There is evidence in animal models that demonstrates negative outcomes on the architecture of the brain including neuronal cell apoptosis and neurodegeneration when exposed to high doses of volatile general anesthetics during critical periods of early neuronal development (41,42). In these animal models, adapted intelligence tests, behavioral assessments, and sensory processing have been shown to be negatively affected when compared to subjects that did not have a general anesthetic exposure (43,44). It is extremely difficult, however, to extrapolate and apply this data to the more complex development of the human brain. There are several major studies that have tried to address this issue by looking at single and multiple exposures to general anesthetics in children under the age of 3 years. Follow-up testing has occurred anywhere from 2 to 18 years after exposure (14-16). The most robust studies on cognitive development after child exposure to anesthesia have come from the following three studies: General Anesthesia Spinal (GAS) trial, Pediatric Anesthesia Neurodevelopment Assessment (PANDA), and Mayo Anesthesia Safety in Kids (MASK). The GAS trial examined single exposures to general anesthetics compared to a neuraxial regional anesthetic technique in children under 3 months of age (14). The primary outcome of this study compared changes in cognition measured through standardized intelligence testing administered at 5 years of age with a secondary outcome measuring cognition at 2 years of age. It is important to recognize that volatile anesthetics are centrally acting medications that have a presumed mechanism of action on the cerebral cortex where neuraxial anesthesia acts directly on the spinal nerves or spinal cord without affecting the cerebral cortex. There was no statistically significant difference between both groups implying that otherwise healthy individuals who had a brief exposure to general anesthesia (less than 1 hour) were not negatively affected in this specific area of neurodevelopment.
Although the GAS trial was able to compare subjects who had relatively similar medical histories, there were a large number of confounding factors that were not included in the analysis. More traditional factors known to affect neurodevelopmental outcomes such as parental influence, familial environment, and socioeconomic status were not entirely accounted for. The PANDA trial addressed this problem by comparing sibling cohorts, which theoretically equalized the non-standardized home environment (15). In this study, patients under the age of three who had a single brief exposure to a general anesthetic were compared to a sibling within 3 years of his or her age. The primary outcome of the study was intelligence measured with a standardized intelligence quotient (IQ) testing and the secondary outcomes looked at differences in broader neurodevelopmental categories such as behavior, executive functioning, and attention. General anesthesia was achieved using both volatile anesthetics and IV anesthetics, which is different from the GAS trial, which only used volatile anesthetics. A regional anesthetic technique including both neuraxial (caudal anesthesia only) and peripheral nerve blocks were sometimes used in conjunction with general anesthesia, but never as the main anesthetic. Similar to the GAS trial, the PANDA trial found no difference in neurocognitive function using primary or secondary outcome measures in single and brief general anesthesia exposures.
The MASK trial addressed questions about repeated exposures to general anesthesia. It looked at children exposed to two or more general anesthetics and compared them to children who had been singly exposed and children who had been unexposed (16). The subjects had all received general anesthesia before the age of three and were evaluated during both preadolescence and adolescence. The mean duration of general anesthesia in the multiple exposure group was 187 minutes, which is almost four times the mean for the single exposure group. As with the other trials, the primary outcome measured was intelligence using a standardized means of testing IQ. The secondary outcomes compared broader neurodevelopmental aspects including motor skills, verbal processing, memory, and behavior. A combination of volatile anesthetics and IV anesthetics were used to obtain general anesthesia, with some patients receiving a regional anesthesia adjunct. This trial found no statistical difference in the primary outcome measuring intelligence. However, there was a statistical difference in two of the secondary outcomes measured, including fine motor skills and processing speeds in the multiple exposed group when compared to the others. However, no difference in these categories was found when the singly exposed group was compared to the unexposed group. From this study, one can infer that exposure to multiple general anesthetics has a negative effect on certain aspects of neurodevelopment.
POA
Infants born prior to 37 weeks are at an increased risk of anesthetic complications. Some articles estimate that premature infants have an in-hospital mortality five times higher than term neonates undergoing non-cardiac surgery (45). Furthermore, the incidence of severe critical events during general anesthesia (complications that led or could have led to major disability or death) are higher in premature neonates compared with their term counterparts (46). The reasons for these are multifactorial, with prematurity being independently associated with multiple systemic manifestations important to the anesthesiologist. One of these considerations is apnea of prematurity. Apnea of prematurity is defined by the American Academy of Pediatrics as cessation of breathing for 20 seconds or longer, or a shorter pause accompanied with bradycardia (<100 beats per minute, cyanosis, or pallor) (47). The consequence of an apneic episode can vary widely depending upon other comorbid conditions and can range from no deviation in vital signs to hypoxia, bradycardia, neurologic complications, and even cardiac arrest (48). In addition to prematurity, other factors such as low birth weight, underlying lung disease, and anemia can also increase the likelihood of POA (49-51). POA decreases significantly by 60 weeks post-conceptual age, which is why elective outpatient surgery is typically performed after this time period. A 2015 meta-analysis demonstrated that the incidence of POA was significantly less in patients receiving spinal anesthesia when other sedatives were not administered (relative risk of 0.53). However, when not adjusted for the additional sedatives, there was no significant difference in POA, partially due to the high technical failure rate of the procedure (2). A secondary aim of the GAS trial evaluated the incidence of POA in neonates who received general anesthesia with a volatile anesthetic versus regional anesthesia with a spinal anesthetic undergoing hernia repair. Apnea was classified either as early, occurring within the first 30 minutes upon arrival to the post-anesthesia care unit, or late, occurring 30 minutes to 12 hours after arrival. There was no difference found in the incidence of both late apnea and overall apneic episodes; however, there was a statistically significant difference found in early apneic episodes. Patients who received regional anesthesia not only had less early apneic episodes, but also required less intervention for resolution of apneic episodes. More specifically, they were less likely to require tactile stimulation, supplemental oxygen, and bag mask ventilation.
Conclusions
Spinal anesthesia appears to be a safe and effective choice for many surgeries of the abdomen, pelvis, and lower extremities in the infant population. The choice of general anesthesia versus spinal anesthesia for healthy, term infants undergoing a single anesthetic does not appear to have a significant effect on neurodevelopment outcomes or POA. However, for patients requiring staged/multiple procedures or with significant comorbidities, utilization of a spinal anesthetic may be a better option. Ultimately, an individualized, careful discussion between parents, anesthesiologists, and surgeons regarding this will elucidate the optimal approach.
Acknowledgments
None.
Footnote
Reporting Checklist: The authors have completed the Narrative Review reporting checklist. Available at https://pm.amegroups.com/article/view/10.21037/pm-24-28/rc
Peer Review File: Available at https://pm.amegroups.com/article/view/10.21037/pm-24-28/prf
Funding: None.
Conflicts of Interest: All authors have completed the ICMJE uniform disclosure form (available at https://pm.amegroups.com/article/view/10.21037/pm-24-28/coif). R.S.I. served as an unpaid editorial board member of Pediatric Medicine from January 2023 to December 2024. R.S.I. declares that he is the founder & CEO of IMG Secrets, a coaching platform for medical graduates. He acknowledges that he has received research funding as a principal investigator (PI) from the Masimo Foundation. This funding is allocated to his institution for research pertaining to the implementation and utilization of the Wake Up Safe database in India. R.S.I. is also the chair of the Society for Pediatric Anesthesia Research Committee (Unpaid). R.S.I. participate on NIH study. The other authors have no conflicts of interest to declare.
Ethical Statement: The authors are accountable for all aspects of the work in ensuring that questions related to the accuracy or integrity of any part of the work are appropriately investigated and resolved.
Open Access Statement: This is an Open Access article distributed in accordance with the Creative Commons Attribution-NonCommercial-NoDerivs 4.0 International License (CC BY-NC-ND 4.0), which permits the non-commercial replication and distribution of the article with the strict proviso that no changes or edits are made and the original work is properly cited (including links to both the formal publication through the relevant DOI and the license). See: https://creativecommons.org/licenses/by-nc-nd/4.0/.
References
- Wiegele M, Marhofer P, Lönnqvist PA. Caudal epidural blocks in paediatric patients: a review and practical considerations. Br J Anaesth 2019;122:509-17. [Crossref] [PubMed]
- Jones LJ, Craven PD, Lakkundi A, et al. Regional (spinal, epidural, caudal) versus general anaesthesia in preterm infants undergoing inguinal herniorrhaphy in early infancy. Cochrane Database Syst Rev 2015;2015:CD003669. [Crossref] [PubMed]
- Batra YK, Lokesh VC, Panda NB, et al. Dose-response study of intrathecal fentanyl added to bupivacaine in infants undergoing lower abdominal and urologic surgery. Paediatr Anaesth 2008;18:613-9. [Crossref] [PubMed]
- Batra YK, Rakesh SV, Panda NB, et al. Intrathecal clonidine decreases propofol sedation requirements during spinal anesthesia in infants. Paediatr Anaesth 2010;20:625-32. [Crossref] [PubMed]
- Frawley G, Ingelmo P. Spinal anaesthesia in the neonate. Best Pract Res Clin Anaesthesiol 2010;24:337-51. [Crossref] [PubMed]
- Tirmizi H. Spinal anesthesia in infants: recent developments. Curr Opin Anaesthesiol 2015;28:333-8. [Crossref] [PubMed]
- Baskin P, Berde C, Saravanan A, et al. Ultrasound-guided spinal anesthesia in infants: a narrative review. Reg Anesth Pain Med 2023;48:608-14. [Crossref] [PubMed]
- Bier A. Experiments regarding the cocainization of the spinal cord. Zentralbl Chir 1899;51:361-9.
- Maltby JR, Hutter CD, Clayton KC. The Woolley and Roe case. Br J Anaesth 2000;84:121-6. [Crossref] [PubMed]
- Vandam LD, Dripps RD. Long-term follow-up of patients who received 10,098 spinal anesthetics. IV. Neurological disease incident to traumatic lumbar puncture during spinal anesthesia. J Am Med Assoc 1960;172:1483-7. [Crossref] [PubMed]
- Abajian JC, Mellish RW, Browne AF, et al. Spinal anesthesia for surgery in the high-risk infant. Anesth Analg 1984;63:359-62. [Crossref] [PubMed]
- Coté CJ, Zaslavsky A, Downes JJ, et al. Postoperative apnea in former preterm infants after inguinal herniorrhaphy. A combined analysis. Anesthesiology 1995;82:809-22. [Crossref] [PubMed]
- Davidson AJ, Disma N, de Graaff JC, et al. Neurodevelopmental outcome at 2 years of age after general anaesthesia and awake-regional anaesthesia in infancy (GAS): an international multicentre, randomised controlled trial. Lancet 2016;387:239-50. Erratum in: Lancet 2016;387:228. [Crossref] [PubMed]
- McCann ME, de Graaff JC, Dorris L, et al. Neurodevelopmental outcome at 5 years of age after general anaesthesia or awake-regional anaesthesia in infancy (GAS): an international, multicentre, randomised, controlled equivalence trial. Lancet 2019;393:664-77. [Crossref] [PubMed]
- Sun LS, Li G, Miller TL, et al. Association Between a Single General Anesthesia Exposure Before Age 36 Months and Neurocognitive Outcomes in Later Childhood. JAMA 2016;315:2312-20. [Crossref] [PubMed]
- Warner DO, Zaccariello MJ, Katusic SK, et al. Neuropsychological and Behavioral Outcomes after Exposure of Young Children to Procedures Requiring General Anesthesia: The Mayo Anesthesia Safety in Kids (MASK) Study. Anesthesiology 2018;129:89-105. [Crossref] [PubMed]
- Walkden GJ, Gill H, Davies NM, et al. Early Childhood General Anesthesia and Neurodevelopmental Outcomes in the Avon Longitudinal Study of Parents and Children Birth Cohort. Anesthesiology 2020;133:1007-20. [Crossref] [PubMed]
- Whitaker EE, Williams RK. Epidural and Spinal Anesthesia for Newborn Surgery. Clin Perinatol 2019;46:731-43. [Crossref] [PubMed]
- Khan A, Eldos Y, AlAnsari KM. Acquired Methemoglobinemia in an Infant: Consequence of Prolonged Application of Eutectic Mixture of Local Anesthetics (EMLA) Cream for Spontaneous Abscess Drainage. Cureus 2022;14:e31304. [Crossref] [PubMed]
- Shahid S, Florez ID, Mbuagbaw L. Efficacy and Safety of EMLA Cream for Pain Control Due to Venipuncture in Infants: A Meta-analysis. Pediatrics 2019;143:e20181173. [Crossref] [PubMed]
- AlSuhebani M, Martin DP, Relland LM, et al. Spinal anesthesia instead of general anesthesia for infants undergoing tendon Achilles lengthening. Local Reg Anesth 2018;11:25-9. [Crossref] [PubMed]
- Hermanns H, Stevens MF, Werdehausen R, et al. Sedation during spinal anaesthesia in infants. Br J Anaesth 2006;97:380-4. [Crossref] [PubMed]
- Jefferson FA, Findlay BL, Handlogten KS, et al. Spinal anesthesia in infants undergoing urologic surgery duration greater than 60 minutes. J Pediatr Urol 2022;18:786.e1-7. [Crossref] [PubMed]
- Ebert KM, Jayanthi VR, Alpert SA, et al. Benefits of spinal anesthesia for urologic surgery in the youngest of patients. J Pediatr Urol 2019;15:49.e1-5. [Crossref] [PubMed]
- Viscomi CM, Abajian JC, Wald SL, et al. Spinal anesthesia for repair of meningomyelocele in neonates. Anesth Analg 1995;81:492-5. [PubMed]
- Williams RK, Abajian JC. High spinal anaesthesia for repair of patent ductus arteriosus in neonates. Paediatr Anaesth 1997;7:205-9. [Crossref] [PubMed]
- Walker BJ, Long JB, Sathyamoorthy M, et al. Complications in Pediatric Regional Anesthesia: An Analysis of More than 100,000 Blocks from the Pediatric Regional Anesthesia Network. Anesthesiology 2018;129:721-32. [Crossref] [PubMed]
- Wolf AR. Effects of regional analgesia on stress responses to pediatric surgery. Paediatr Anaesth 2012;22:19-24. [Crossref] [PubMed]
- Williams RK, Adams DC, Aladjem EV, et al. The safety and efficacy of spinal anesthesia for surgery in infants: the Vermont Infant Spinal Registry. Anesth Analg 2006;102:67-71. [Crossref] [PubMed]
- Kiechl-Kohlendorfer U, Unsinn KM, Schlenck B, et al. Cerebrospinal fluid leakage after lumbar puncture in neonates: incidence and sonographic appearance. AJR Am J Roentgenol 2003;181:231-4. [Crossref] [PubMed]
- Allee JI, Goins KM, Berde CB, et al. A case of cerebrospinal fluid leak in an infant after spinal anesthesia. J Clin Anesth 2013;25:217-9. [Crossref] [PubMed]
- Kokki H, Hendolin H, Turunen M. Postdural puncture headache and transient neurologic symptoms in children after spinal anaesthesia using cutting and pencil point paediatric spinal needles. Acta Anaesthesiol Scand 1998;42:1076-82. [Crossref] [PubMed]
- Easley RB, George R, Connors D, et al. Aseptic meningitis after spinal anesthesia in an infant. Anesthesiology 1999;91:305-7. [Crossref] [PubMed]
- Imbelloni LE, Vieira EM, Sperni F, et al. Spinal anesthesia in children with isobaric local anesthetics: report on 307 patients under 13 years of age. Paediatr Anaesth 2006;16:43-8. [Crossref] [PubMed]
- Dohi S, Seino H. Spinal anesthesia in premature infants: dosage and effects of sympathectomy. Anesthesiology 1986;65:559-61. [Crossref] [PubMed]
- Sola C, Hertz L, Bringuier S, et al. Spinal anaesthesia in neonates and infants: what about the cerebral oxygen saturation? Br J Anaesth 2017;119:964-71. [Crossref] [PubMed]
- McCann ME, Withington DE, Arnup SJ, et al. Differences in Blood Pressure in Infants After General Anesthesia Compared to Awake Regional Anesthesia (GAS Study-A Prospective Randomized Trial). Anesth Analg 2017;125:837-45. [Crossref] [PubMed]
- Dohms K, Hein M, Rossaint R, et al. Inguinal hernia repair in preterm neonates: is there evidence that spinal or general anaesthesia is the better option regarding intraoperative and postoperative complications? A systematic review and meta-analysis. BMJ Open 2019;9:e028728. [Crossref] [PubMed]
- Kachko L, Simhi E, Freud E, et al. Impact of spinal anesthesia for open pyloromyotomy on operating room time. J Pediatr Surg 2009;44:1942-6. [Crossref] [PubMed]
- Ing C, Sun LS, Friend AF, et al. Adverse Events and Resource Utilization After Spinal and General Anesthesia in Infants Undergoing Pyloromyotomy. Reg Anesth Pain Med 2016;41:532-7. [Crossref] [PubMed]
- Ing C, DiMaggio C, Whitehouse A, et al. Long-term differences in language and cognitive function after childhood exposure to anesthesia. Pediatrics 2012;130:e476-85. [Crossref] [PubMed]
- Jevtovic-Todorovic V, Hartman RE, Izumi Y, et al. Early exposure to common anesthetic agents causes widespread neurodegeneration in the developing rat brain and persistent learning deficits. J Neurosci 2003;23:876-82. [Crossref] [PubMed]
- Paule MG, Li M, Allen RR, et al. Ketamine anesthesia during the first week of life can cause long-lasting cognitive deficits in rhesus monkeys. Neurotoxicol Teratol 2011;33:220-30. [Crossref] [PubMed]
- Raper J, Alvarado MC, Murphy KL, et al. Multiple Anesthetic Exposure in Infant Monkeys Alters Emotional Reactivity to an Acute Stressor. Anesthesiology 2015;123:1084-92. [Crossref] [PubMed]
- Lillehei CW, Gauvreau K, Jenkins KJ. Risk adjustment for neonatal surgery: a method for comparison of in-hospital mortality. Pediatrics 2012;130:e568-74. [Crossref] [PubMed]
- Habre W, Disma N, Virag K, et al. Incidence of severe critical events in paediatric anaesthesia (APRICOT): a prospective multicentre observational study in 261 hospitals in Europe. Lancet Respir Med 2017;5:412-25. Erratum in: Lancet Respir Med 2017;5:e19 Erratum in: Lancet Respir Med 2017;5:e22. [Crossref] [PubMed]
- Eichenwald ECCommittee on Fetus and Newborn, American Academy of Pediatrics. Apnea of Prematurity. Pediatrics 2016; [Crossref] [PubMed]
- Gharavi-Fard M. Apneas in Infants with Postconceptional Age bellow 60 Weeks Undergoing Herniorrhaphy. Iran J Pediatr 2014;24:179-83. [PubMed]
- O'Reilly M, Sozo F, Harding R. Impact of preterm birth and bronchopulmonary dysplasia on the developing lung: long-term consequences for respiratory health. Clin Exp Pharmacol Physiol 2013;40:765-73. [Crossref] [PubMed]
- del Cerro MJ, Sabaté Rotés A, Cartón A, et al. Pulmonary hypertension in bronchopulmonary dysplasia: clinical findings, cardiovascular anomalies and outcomes. Pediatr Pulmonol 2014;49:49-59. [Crossref] [PubMed]
- Zagol K, Lake DE, Vergales B, et al. Anemia, apnea of prematurity, and blood transfusions. J Pediatr 2012;161:417-421.e1. [Crossref] [PubMed]
Cite this article as: Garcia K, Julian N, Spiro J, McClung H, Iyer RS. Infant spinal anesthesia: a narrative review. Pediatr Med 2025;8:3.