Limitations of adaptive physiology: are normal values really normal in the critically ill child?
Introduction
Critical illness reflects an interplay between the pathological processes of disease and the physiological processes of adaptation. The adaptive stress response is a bundle of behavioral, physiological and cellular responses that aim to adapt the organism to internal or external changes. This adaptation aims to preserve cellular integrity according to a functional hierarchy of organ systems that are vital to the survival of an organism (1-3).
Decompensation occurs if the pathological processes prevail over the stress response (an inadequate response), or over-drive the response to the point of it becoming maladaptive (an over-active response). For example, loss of local vascular tone without a compensatory rise in cardiac output can lead to an ischemic stroke; however, an exaggerated blood pressure (BP) response with local hyperemia can lead to hemorrhagic transformation.
This notion that critical illness is a condition of stress-related decompensation has evolved over centuries. Hippocrates described health as a balance of four basic humors (blood, black bile, yellow bile, and phlegm) and illness as a loss of the equilibrium (4). Claude Bernard asserted that complex organisms could maintain their internal environment (milieu interieur) fairly constant in the face of challenges from the external world (5). Walter Bradford Cannon adapted this concept and coined the term “homeostasis,” which focused on the ability to maintain a steady state within an organism. He believed that illness happens when homeostatic systems fail to keep physiology within normal ranges. Cannon also described the fright-fight-flight response where, in the face of a stressor, blood flow increases to the “essential” organs-brain, heart, lung, and skeletal muscles at the expense of the “non-essential” organs such as the digestive system (6). David Cuthbertson explored the metabolic changes in the body as a response to a stressor, and he was the first to describe the ebb (shock) and flow (post-shock) phases of post-traumatic stress, which had been used for many years to guide the resuscitation of patients with shock (7). Later physiologists such as Selye and Laborit described the possible deleterious effects that the adaptive mechanisms to physiologic stress may have (8,9). Subsequently, Singer et al. described the biphasic inflammatory, immune, hormonal, and metabolic response to critical illness. The acute phase constitutes an upregulation in mitochondrial and metabolic activity stemming from an abrupt rise in stress hormones. Thereafter, functional mechanisms take over in an attempt to ensure cell survival. There is a reduction in energy production, metabolic rate and normal cellular processes from secondary changes in the endocrine profile and severe inflammation, leading to multi-organ dysfunction or failure (10).
The management of critical illness is centered on preventing the loss of, or reinstating thereafter, physiologic homeostasis. Concepts such as “the golden hour” of resuscitation and early goal directed therapy aim to restore physiology and prevent organ dysfunction (11-13). Intensive care focuses on maintaining the balance between oxygen delivery and consumption, based on the Guytonian models of shock (14). Interventions are aimed at manipulating physiological variables such as gas exchange and BPs. We infer that by monitoring and improving parts of a system such as oxygen delivery, we can optimize the performance of the whole. In his much-loved satire essay Alice in intensiveland, Bartlett described the intensive care unit (ICU) as a place where “…technology triumphs over disease…. Here we can measure everything. With measurements, we can do calculations. With calculations, we can do logarithms. With logarithms, we can solve any clinical problem.” (15)
Much as decompensation occurs with an inadequate or over-active stress response, intensive care interventions need to find an optimum point between under and over-treatment. This optimum may not always be a state of normal physiology, as defined during a state of health, but may need to mimic the adaptive physiology of compensated critical illness. Whilst this remains difficult in adult physiology, in children this is even more so.
The monitoring of physiology can be challenging in children due to limitations of size and technology and as normal and adaptive physiology develops with age, even definitions of normal ranges are conflicting (16-18). These definitions will also vary considerably in children with specific co-morbidities or underlying pathologies, such as those with cardiac dysfunction or congenital heart disease. The specific issues pertaining to critically ill cardiac patients are outside of the scope of this review.
The intensive care strategy of monitor-manipulate-evaluate cycle is designed to empirically achieve the balance between under and over treatment. Under treatment may result in morbidity and mortality, while the harms of over diagnosis and overtreatment are becoming more apparent in modern practice. This has led to the “Choosing Wisely” campaign, in which medical societies have identified many tests, medications, and treatments that are misused (19,20). Life supporting therapy in ICUs has been increasingly offered to patients with poor long-term prognoses, including those with multi-organ failure or poor functional status at baseline. This has become a worrying trend as it can lead to futile, disproportionate or inappropriate care (21).
In this review we aim to evaluate the available evidence to guide interventions in the children on the ICU. We concentrate on 6 parameters universally measured by the bedside on PICUs: oxygen saturation (SpO2), heart rate (HR), BP, hemoglobin, temperature and glucose. We present the balance of evidence, question the dogma of accepted practice, and highlight the research priorities to improve the care of critically ill children.
SpO2
Maintaining adequate tissue oxygenation is a key goal in the management of critically ill children. The most common form of organ support provided to children in the ICU setting is invasive ventilation with supplemental oxygen in order to maintain safe levels of SpO2, and partial pressure of oxygen (PaO2) (22). Both supplemental oxygen therapy and hypoxia are associated with adverse effects in critically ill patients. It is known that hypoxia leads to cell death, while hyperoxia can lead to cell injury through the formation of reactive oxygen species (23,24), though the “ideal” range for PaO2 and SpO2 in critically ill children has yet to be defined. Two studies have looked at the relationship between PaO2 on admission to pediatric intensive care unit (PICU) and mortality and both found a “U-shaped” relationship between PaO2 and mortality (25,26). Raman et al. surveyed members of the UK Paediatric Intensive Care Society (PICS) on practices around oxygen delivery and found that practices around oxygen administration and targets varied considerably with a majority of respondents working in units without oxygen weaning protocols (27). In a subsequent single center study looking at 7,352,388 SpO2 values from 227 children admitted to PICU. Ray et al. demonstrated that current PICU practice is biased towards a liberal oxygenation strategy well above recommended targets, even in children with low PaO2 to fractional inspired oxygen (FiO2) ratios (PF ratios) (28).
Certain disease states have management guidelines, such as the ARDSnet protocol, that clearly prescribe the use of conservative oxygenation targets (29,30). This is centered on the fact that there are deleterious effects of using ventilation strategies that apply high ventilation pressures, volumes and inspired fractions of FiO2 (30). In children with acute respiratory distress syndrome (ARDS) or severe acute lung injury (ALI), SpO2 target should be 88–92% if on a positive end-expiratory pressure (PEEP) of ≥10 cmH2O or 92–97% if PEEP is <10 cmH2O (31). A few studies have looked at the negative consequences of hyperoxia in certain pathologies. In adult patients, hyperoxia has been independently associated with increased mortality in patients following cardiac arrest (32,33), post-stroke (34) and in respiratory failure admitted to ICU (35). In a recent systematic review, Chu et al. found that the provision of supplemental oxygen in acutely ill adults (regardless of the etiology of their disease) who had normal SpO2 before the initiation of oxygen therapy had increased mortality (36). In addition, the BMJ has published recommendations advocating for the conservative use of supplemental oxygen (37) In pediatric patients, this association has been described in patients with congenital heart disease requiring extra-corporeal life support (38). In a randomized, equivalence trial, Cunningham et al. showed that managing infants with bronchiolitis using a SpO2 target of 90% was safe and as effective as a 94% target and led to quicker discharge from hospital (39). In preterm neonates, adverse consequences associated with the liberal use of oxygen have long been recognized (since the early 1940s). Many studies have been designed to try to establish the optimal SpO2 levels in this population. Askie et al. published a systematic review exploring the effects of conservative versus liberal SpO2 ranges on mortality or major morbidities in the extremely preterm infants. They included 5 trials in their meta-analysis, and found no significant effect on the composite outcome of mortality or major disability or on major disability alone, but they did find an increased risk of death (by 28 per 1,000 infants treated) in the group with lower SpO2 targets (40).
Further work is ongoing in an attempt to delineate the “ideal” target range, which balances the harms and benefits of supplemental oxygen therapy in critically ill pediatric patients. Earlier this year, Peters et al. published the results of a randomized multi-center pilot trial looking to assess the feasibility of a large-scale trial looking at comparing a liberal oxygenation strategy (SpO2 >94%) versus a conservative oxygenation strategy (SpO2 88–92%). They found no significant between-group differences in length of stay, duration of organ support or mortality and concluded that a definitive clinical trial of peripheral SpO2 targets would be possible in the PICU population (22).
HR
HR is a vital determinant of cardiac output and thus is one of the key variables that are closely monitored in the critical care setting. It serves to monitor the impact of interventions, the response to treatment and to identify the deteriorating child early. A patient’s HR is affected by a number of factors, many of which are manipulated in the critical care setting. HR fluctuates significantly with temperature, pain, blood loss, medications and many other factors that are related to any stay in the ICU. In the context of these factors, the interpretation of an individual patient’s HR is challenging. What constitutes a “normal” HR is not well defined, especially in the critically ill child.
In 2011, Fleming et al. published a systematic review of 69 observational studies looking at defining “normal” HR and respiratory rate in healthy children birth to 18 years (41). They found that their values showed striking disagreement when compared to previously published ranges primarily based on clinical consensus. Subsequent studies, by Daymont & Bonafide et al., sought to define reference ranges for ill children admitted to inpatient wards and to understand the impact of temperature on HR ranges (42,43). They were able to derive and validate percentile curves for HR and respiratory rate in hospitalized children, finding that their ranges also differed from existing reference ranges and commonly used early warning score values (42). In 2017, Eytan et al. published the first and to our knowledge only study reporting the HR centile curves and distributions by age in critically ill pediatric patients. They found that the HR values in these children were markedly higher than in healthy patients and children admitted to inpatient wards. When compared to commonly referenced values published in the Pediatric Advanced Life Support (PALS) guidelines, a large proportion of children after the age of 2 years exhibited values well above what would be considered abnormal by these guidelines (16). These data did not take into account diagnosis, procedures, sedation, vasoactive agents, ventilation status or other potential modifiers and thus should not be used as normative reference ranges.
In addition, HR is an important variable used in a variety of clinical guidelines. One of the first goals of the first hour of resuscitation in sepsis is to “maintain or restore threshold HR” (44). Threshold HR is defined as per the Pediatric Risk of Mortality score (PRISM) score and values higher than these are associated with increased mortality in critically ill children (infants HR <90 beats/min or HR >160 beats/min; children HR <70 beats/min or HR >150 beats/min) (45). Furthermore, HR is a variable being used in the development of predictive modelling using machine learning. In the neonatal population, HR characteristic monitoring (decreased variability and transient decelerations) has been used to identify infants at high risk of sepsis, urinary tract infection (UTI) and death, and predictive modelling has been developed to aid in early detection of sepsis (46,47). Earlier this year, Kamaleswaran et al. successfully used artificial intelligence to predict the onset of severe sepsis using HR and other physiologic markers in critically ill children (48).
Concrete definitions of what constitutes a “normal” HR range for age in critically ill children are urgently needed, and further work is needed to understand how best to utilize this physiologic variable in identifying, predicting and treating disease in the critically ill.
BP
BP is an essential marker of hemodynamic stability in critically ill children. Systolic blood pressure (SBP) is associated with mortality and is a factor used in various mortality prediction models such as the Paediatric Index of Mortality (PIM) and the PRISM (49,50). In 1997, during the development of the PIM score, Shann et al. found that when SBP was plotted against mortality there was a clear U-shaped curve with a nadir at an SBP of 120 mmHg (51) Thus, maintaining a “normal” BP and avoiding hypotension are among the fundamental tenets of managing sick children (52), though the optimal reference values and BP targets remain largely unknown.
Reference values have been gathered using auscultatory data from healthy children, the purpose of which was to define hypertension and not hypotension (53,54). The US National Institute of Health task force data provide the gold-standard reference ranges for the 50th and 95th centile values in healthy children and infants (53,55). Using these data, others have estimated 5th percentile values assuming normal distribution (17). The definitions of 5th centile SBP norms for children vary significantly between different reference sources such as PALS, Advanced Paediatric Life Support (APLS) and the Revised Consensus Conference data (54). This multitude of definitions has the potential to cause confusion at the bedside. Furthermore, there are no specific reference ranges available for pediatric intensive care patients. Abdelrazeq et al. found that compared to population-based normal values, SBP measurements in children admitted to the PICU showed that age distributed BP values were significantly different to the normative values gathered from healthy children. Overall, critically ill children, and especially younger children, had higher BPs (either maintained or tolerated) (56).
The method used to monitor BP is also important. Ray et al. did a comparative analysis of invasive arterial blood pressure (IABP) and non-invasive oscillometric blood pressure (NIBP) measurements, finding that NIBP gave lower readings for both mean and diastolic BP when compared to corresponding IABP (57). These data suggest a potential for over-treatment of “hypotension,” especially as fewer critically ill children are being managed with IABP monitoring.
Just as there is a definite lack of consensus on what should be considered “normal” BP, there is a paucity of data to guide clinical management as to what levels should be targeted in different clinical scenarios. There is evidence to suggest that outcomes are worse in children who experience hypotension, defined as SBP <5th centile, following a cardiac arrest (58,59) but no other evidence-based data are available to guide practice at the bedside concretely. Prospective studies are urgently needed to delineate both normative values and clear BP targets in critically ill children.
Hemoglobin
Oxygen delivery is critical in maintaining normal metabolism. It is the product of total blood flow to the tissues and the oxygen content in the blood, which is mostly bound to hemoglobin. In the critically ill, oxygen delivery can be impaired by several mechanisms and Hemoglobin levels are often monitored and targeted. Both anemia and red blood cell transfusion are associated with increased mortality (60). Red blood cell transfusions can be lifesaving in certain conditions such as active bleeding, severe anemia or bone marrow failure. Transfusions are also indicated for other critical conditions such as shock or respiratory failure to maintain or restore the oxygen-carrying capacity of the blood to avoid tissue hypoxia.
Anemia is prevalent in critically ill children; with 74% having low hemoglobin levels with PICU stays longer than two days. Almost half of these children receive at least one red blood cell transfusion during their admission (61). However, transfused red blood cells are vulnerable to storage lesions that can reduce their ability to bring oxygen to the cells. Both impaired membrane deformability that compromises red blood cell passage into the microcirculation and acute microvascular dysregulation induced by alterations in the nitric oxide signaling have been described (62,63). Transfusions also carry risks such as infections, transfusion-associated acute lung injury, transfusion-associated circulatory overload, transfusion-associated respiratory dysfunction, and allergic reaction. Recent studies have demonstrated an association between transfusions and immune depression (64) and the development of ICU-delirium (65). Even though a red blood cell transfusion can increase blood oxygen content, it is not clear that stored red blood cell can improve the delivery of oxygen to the tissues as expected and considering the potential risks, a cautious transfusion strategy is warranted in critically ill children.
Clinical trials have studied the implications of liberal versus restrictive hemoglobin transfusion strategies in both adult and pediatric populations. Lacroix et al found that in stable, critically ill children, using a restrictive strategy with a hemoglobin threshold of 7 g per deciliter as compared to a liberal strategy, resulted in a 96% reduction in the number of patients who received a transfusion and a 44% decrease in the total number of red blood cell transfusions administered without increasing the rates of multi-organ failure (60,66,67).
Respiratory distress has been shown to be associated with increased mortality in the context of severe anemia. Marsh et al. looked at 1,866 children admitted to a Kenyan hospital with a primary diagnosis of malaria and found that the mortality rate was 1.3% if severe anemia (hemoglobin <5 g per deciliter) was present without respiratory distress and increased to 22.7% if severe respiratory distress was also present (68). Recently the Pediatric Critical Care Transfusion and Anemia Expertise Initiative (TAXI), in collaboration with the Pediatric Critical Care Blood Research Network (BloodNet), and the Pediatric Acute Lung Injury and Sepsis Investigators (PALISI) Network initiative addressed this patient group specifically and published their recommendations on red blood cell transfusions in critically ill children with acute respiratory failure. Their recommendations also support a restrictive strategy of red blood cell transfusion for children who are hemodynamically stable. They advise transfusing when the hemoglobin level is lower than 5 g per deciliter and not to transfuse when it is above 7 g per deciliter in stable children. They did not provide a strategy in children with hemodynamic instability, severe hypoxia or hemoglobin levels between 5–7 g per deciliter (61). The same group reviewed the indications for red blood cells transfusion in children requiring extra-corporeal support (including Extracorporeal Membrane Oxygenation, Ventricular Assist Devices, and Renal Replacement Therapy), and found that there is currently insufficient evidence to recommend specific red blood cell transfusion thresholds in this group (69). Therefore, the recommendation remains that transfusions should be “targeted to symptomatic patients only” (70).
Although not involved with oxygen delivery, a similar approach may also be applicable to transfusion of other blood products. Recently, in the neonatal population, Curley et al. conducted a multi-center randomized trial comparing a low (platelets <25,000 per cubic millimeter) versus a high (platelets <50,000 per cubic millimeter) transfusion threshold. In the 660 premature (<34 weeks) neonates studied, they found that the infants assigned to the high threshold group had significantly higher rate of death or major bleeding (71). Further studies are needed to provide recommendations for platelet transfusion strategies in PICU patients and hemoglobin transfusion thresholds in critically ill children requiring extra-corporeal support and for children with hemoglobin levels between 5–7 g per deciliter.
Temperature
Temperature is a much-debated topic in the management of critically ill children. What constitutes an “ideal” body temperature has not been clearly defined and likely varies depending on the underlying diagnosis. Targeted normothermia is a common neuroprotective strategy in the management of children with specific diagnoses, including post-cardiac arrest and traumatic brain injury, yet the evidence does not suggest a benefit with therapeutic hypothermia and in some cases showed harm (72). In contrast, targeted hypothermia is the standard of care for term, and late preterm neonates with hypoxic-ischemic encephalopathy as the studies have demonstrated reduced mortality and severe disability with the use of this intervention (73).
The role that fever plays in critical illness and outcomes of critically ill children remains unclear. Fever plays an essential role in the host response to infection and is usually considered to be a good adaptive response, while hypothermia is deemed to be maladaptive in the setting of infection (74). Despite this, there is a propensity to treat fever in pediatrics. A survey of 462 pediatric intensive care medical and nursing staff in the UK showed a tendency towards the treatment of fever at a median temperature threshold of 38 °C (75).
Anti-pyretic medications, such as paracetamol, are commonly used in the PICU setting both for analgesia and treatment of fever. It has been shown that paracetamol impairs patients’ immune response to vaccination (76) and causes a delay in the time to crusting of chickenpox (77). In addition, children with malaria experience a longer time to resolution of parasitemia when treated with paracetamol (78). In 2017, Ray et al. published a prospective observational study in children looking at the hemodynamic consequences of paracetamol administration and found that there was a significant decrease in mean arterial BP, more pronounced in those with fever at baseline (79).
In large adult studies, it has been shown that the use of antipyretics in critically ill patients with sepsis is associated with higher mortality (80) and that patients with infection and a febrile response have a lower adjusted odd of death compared to those without fever (81). There is a lack of evidence in pediatric patients. One small trial in PICU patients found that fever after 48 hours of admission or a secondary episode of fever was independently associated with prolonged PICU stay and length of ventilation (82). One very small study of 12 patients looked at resting energy expenditure during febrile illness and failed to find a consistent alteration in metabolic rate in these infants (83). Despite this, it is generally considered prudent to treat fever in patients in whom the high-energy burden may pose a significant physiologic challenge (74,83), such as in children with congenital heart disease, uncontrolled septic shock or traumatic brain injury. There remain many questions surrounding the harms and benefits of fever and antipyretic use in critically ill children. Two ongoing studies [FEVER study (an epidemiological observational study) and the FEVER pilot trial (a comparison of a liberal vs. conservative fever management thresholds)] are anticipated to provide more data and hopefully shed light on the current questions and controversies.
Glucose
Glucose homeostasis is often impaired in critical illness. Hyperglycemia is part of the stress response and is common, occurring in 45–86% of critically ill children, depending on what definition is used (84). Possible mechanisms include impaired glucose metabolism, relative insulin deficiency, peripheral insulin resistance, and medication administration (85). Hyperglycemia has been associated with increased duration of hospital stay, organ failure and death in children (85-87). Hypoglycemia, either spontaneous or iatrogenic, is less frequent but still occurs in 2.2% to 9.7% of PICU patients and has been associated with worse neurologic outcomes, increased length of ICU stays and mortality (88,89).
In 2001, van den Berghe et al. published the results of a randomized controlled trial comparing intensive insulin therapy [blood glucose (BG) between 4.4–6.1 mmol/L per liter] and conventional therapy (insulin infusion for BG >12mmol/L, followed by target range of BG 10.0–11.1 mmol per liter) in critically ill adults. They found that those in the intensive therapy group were less likely to need prolonged mechanical ventilation and ICU stay and had lower mortality (90). This landmark trial led to a number of pediatric and adult studies that further explored the relationship between tight glucose control and outcomes. In 2009, the international NICE-SUGAR trial, found that intensive glucose control increased mortality compared to a conventional approach in adult ICU patients (91).
In 2009, the first pediatric RCT was published comparing two target thresholds (BG 2.8–4.4 mmol per liter versus insulin to prevent BG from exceeding 11.9 mmol per liter) in 700 critically ill children and found that targeting the lower threshold reduced the duration of PICU stay but caused a much higher rate of hypoglycemia (BG ≤2.2 mmol per liter in 25% vs. 1%) and severe hypoglycemia (BG ≤1.7 mmol per liter in 5% vs. 1%) in the low target group (92). Agus et al. studied 980 children following surgery with cardiopulmonary bypass comparing tight glycemic control (BG 4.4–6.1 mmol per liter) versus standard care. They found that tight control was possible with less frequent episodes of severe hypoglycemia (BG <2.2 mmol per liter in 3% vs. 1%) than previously reported but the outcomes between the groups did not differ significantly (93). Subsequently, the CHiP trial randomized 1,369 PICU patients to a tight glycemic control group (4.0–7.0 mmol per liter) versus a conventional group (target BG <12.0 mmol per liter) and found no significant effect of tight glycemic control on clinical outcomes, though the 12-month costs were lower in the tight control group (94). In 2017, the HALF-PINT trial explored this question again, comparing two BG target ranges (BG 4.4–6.1 mmol per liter vs. BG 8.3–10.0 mmol per liter) in PICU patients from 35 centers. The trial was stopped early as it was deemed to have a low likelihood of benefit and evidence of the possibility of harm (non-significantly higher mortality, increased infection and severe hypoglycemia) in the lower threshold group (95). Strict glycemic control has been extensively studied and has not been shown to confer any significant benefits and is associated with potential harm. Thus, a cautious approach is warranted when considering insulin therapy in critically ill patients.
Another such therapy that has come under scrutiny is the use of parenteral nutrition (PN). The PEPaNIC trial compared early (within 24 hours of admission) versus late (day 8 of admission) initiation of PN in 1,440 critically ill children and found that the late-parenteral-nutrition group had fewer new infections, shorter duration of mechanical ventilation and earlier live discharge from PICU and hospital (96). Thus, the timing of initiation of PN warrants a thoughtful approach and further studies may be needed to outline the optimal strategy in critically ill children.
Table 1 summarizes the balance of evidence and the accepted clinical practices for the physiologic parameters reviewed.
Table 1
Physiologic variable | Is normalization evidence based-standard of care? | Summary of the literature |
---|---|---|
SpO2 | No | (I) Target SpO2 of ≥90% for infants with bronchiolitis (38) and 88–92% in children with ARDS or severe acute lung injury on a PEEP of ≥10 cmH2O (30); |
HR | No (unknown) | (I) Normative values are unknown in critically ill children; |
BP | No | (I) Normative values are unknown in critically ill children; |
Hgb/platelets | No | (I) Critically ill children who are hemodynamically stable should be transfused when Hgb is <5 g/dL and not be transfused when it is >7 g/dL (61); |
Temperature | No | (I) Targeted normothermia is indicated for neuro-protection in patients with traumatic brain injury and post cardiac arrest (72); |
Glucose | No | (I) Hyperglycemia has been associated with increased length of ICU stay, organ failure and mortality (90,91); |
SpO2, oxygen saturation; HR, heart rate; BP, blood pressure; Hgb, hemoglobin; ARDS, acute respiratory distress syndrome; PEEP, positive end-expiratory pressure; PICU, pediatric intensive care unit.
Conclusions
The care of critically ill patients involves a balance between the potential harms and benefits of lifesaving interventions and therapies. The goal is to find the optimum point between under and over treatment in an attempt to restore physiologic homeostasis (Figure 1).
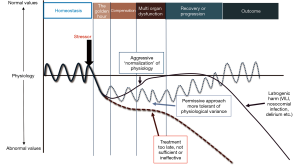
Even the basic vital signs lack clear normative data in critically ill pediatric patients and many of the treatment guidelines are not underpinned by rigorous pediatric evidence but rather expert consensus. The goals of intensive care should be fluid with consideration for the pathology of the disease and the adaptive physiology of the patient.
Achieving this balance between under and over treatment applies across a wide spectrum of disease severity. In the most severe states of disordered homeostasis, there may be a complete loss of control that no physiologic response can fully contain, such as in cardiac or respiratory arrest. Management of these situations must be centered on vigorous resuscitation to reinitiate oxygen delivery. Even in situations during which cardiopulmonary resuscitation (CPR) is being provided, the literature suggests that over treatment may cause harm and may impede the resuscitation efforts. For example, studies have shown that an increased ventilation rate during CPR leads to higher mean intrathoracic pressure which in turn reduces venous return, cardiac output, coronary perfusion pressure and aortic BP, thereby decreasing the chances of attaining return of spontaneous circulation (97-99).
Furthermore, in clinical situations where despite best efforts to reestablish physiologic homeostasis a favorable outcome is not possible, it is critical to quickly recognize that the goals of care need to shift towards quality end of life care to serve the best interests of our patients and their families. In circumstances where end of life care may be more appropriate, “lifesaving” interventions become ethically complex and may be perceived as excessive or inappropriate by the medical staff or the patient’s families, which may lead to moral distress and burnout. Recent data has shown that situations, in which physicians perceived the care provided to be excessive, were predictive of poor outcomes, including death (100) suggesting that improving the ethical climate in the ICU may ameliorate the quality of end of life care and perhaps improve the moral well-being of the medical staff.
In this review, we have focused on six commonly monitored and manipulated physiologic variables and presented the evidence behind current recommendations and highlighted some of the insufficiencies in the definitions of normative values and commonly accepted practices. The dogma of ICU medicine must continue to be challenged, to ensure that ultimately, we are providing the best care for critically ill children.
Acknowledgments
Funding: This study received no direct funding but was supported by the National Institute for Health Research Great Ormond Street Hospital Biomedical Research Centre. The views expressed are those of the authors and not necessarily those of the NHS, the NIHR or the UK Department of Health.
Footnote
Conflicts of Interest: All authors have completed the ICMJE uniform disclosure form (available at http://dx.doi.org/10.21037/pm.2018.12.03). MP serves as an unpaid editorial board member of Pediatric Medicine from Jul 2018 to Jun 2020. SR received no direct funding but The employing institution (Great Ormond Street Hospital NHS Foundation Trust) was supported by the National Institute for Health Research Great Ormond Street Hospital Biomedical Research Centre. The views expressed are those of the authors and not necessarily those of the NHS, the NIHR or the UK Department of Health. The other authors have no conflicts of interest to declare.
Ethical Statement: The authors are accountable for all aspects of the work in ensuring that questions related to the accuracy or integrity of any part of the work are appropriately investigated and resolved.
Open Access Statement: This is an Open Access article distributed in accordance with the Creative Commons Attribution-NonCommercial-NoDerivs 4.0 International License (CC BY-NC-ND 4.0), which permits the non-commercial replication and distribution of the article with the strict proviso that no changes or edits are made and the original work is properly cited (including links to both the formal publication through the relevant DOI and the license). See: https://creativecommons.org/licenses/by-nc-nd/4.0/.
References
- Cuesta JM, Singer M. The stress response and critical illness: a review. Crit Care Med 2012;40:3283-9. [Crossref] [PubMed]
- De Cosmi V, Milani GP, Mazzocchi A, et al. The Metabolic Response to Stress and Infection in Critically Ill Children: The Opportunity of an Individualized Approach. Nutrients 2017;9. [PubMed]
- Chrousos GP, Gold PW. The concepts of stress and stress system disorders. Overview of physical and behavioral homeostasis. JAMA 1992;267:1244-52. [Crossref] [PubMed]
- Hippocrates. On the Sacred Disease. Available online: http://classics.mit.edu/Hippocrates/sacred.html
- Bremier T. Leçons sur les phénomènes de la vie communs aux animaux et aus végétaux. Paris: Baillère; 1878.
- Cannon W. The wisdom of the body. New York: WW Norton; 1932.
- Wilmore DW. From Cuthbertson to fast-track surgery: 70 years of progress in reducing stress in surgical patients. Ann Surg 2002;236:643-8. [Crossref] [PubMed]
- Selye H. Forty years of stress research: principal remaining problems and misconceptions. Can Med Assoc J 1976;115:53-6. [PubMed]
- Laborit H. General biological basis of reaction to aggression. Agressologie 1972;13:1-54. [PubMed]
- Singer M, De Santis V, Vitale D, et al. Multiorgan failure is an adaptive, endocrine-mediated, metabolic response to overwhelming systemic inflammation. Lancet 2004;364:545-8. [Crossref] [PubMed]
- Carcillo JA, Davis AL, Zaritsky A. Role of early fluid resuscitation in pediatric septic shock. JAMA 1991;266:1242-5. [Crossref] [PubMed]
- Wheeler DS. Is the “golden age” of the “golden hour” in sepsis over? Crit Care 2015;19:447. [Crossref] [PubMed]
- Rivers E, Nguyen B, Havstad S, et al. Early goal-directed therapy in the treatment of severe sepsis and septic shock. N Engl J Med 2001;345:1368-77. [Crossref] [PubMed]
- Guyton AC, Crowell JW. Dynamics of the heartin shock. Fed Proc 1961;20:51-60. [PubMed]
- Bartlett RH. Alice in intensiveland. Being an essay on nonsense and common sense in the ICU, after the manner of Lewis Carroll. Chest 1995;108:1129-39. [Crossref] [PubMed]
- Eytan D, Goodwin AJ, Greer R, et al. Heart Rate and Blood Pressure Centile Curves and Distributions by Age of Hospitalized Critically Ill Children. Front Pediatr 2017;5:52. [Crossref] [PubMed]
- Haque IU, Zaritsky AL. Analysis of the evidence for the lower limit of systolic and mean arterial pressure in children. Pediatr Crit Care Med 2007;8:138-44. [Crossref] [PubMed]
- Ramgopal S, Elmer J, Escajeda J, et al. Differences in Prehospital Patient Assessments for Pediatric Versus Adult Patients. J Pediatr 2018;199:200-5.e6. [Crossref] [PubMed]
- Casarett D. The Science of Choosing Wisely--Overcoming the Therapeutic Illusion. N Engl J Med 2016;374:1203-5. [Crossref] [PubMed]
- Carter SM, Rogers W, Heath I, et al. The challenge of overdiagnosis begins with its definition. BMJ 2015;350:h869. [Crossref] [PubMed]
- Kompanje EJ, Piers RD, Benoit DD. Causes and consequences of disproportionate care in intensive care medicine. Curr Opin Crit Care 2013;19:630-5. [PubMed]
- Peters MJ, Jones GAL, Wiley D, et al. Conservative versus liberal oxygenation targets in critically ill children: the randomised multiple-centre pilot Oxy-PICU trial. Intensive Care Med 2018;44:1240-8. [Crossref] [PubMed]
- Helmerhorst HJ, Schultz MJ, van der Voort PH, et al. Bench-to-bedside review: the effects of hyperoxia during critical illness. Crit Care 2015;19:284. [Crossref] [PubMed]
- Magder S. Reactive oxygen species: toxic molecules or spark of life? Crit Care 2006;10:208. [Crossref] [PubMed]
- Raman S, Prince NJ, Hoskote A, et al. Admission PaO2 and Mortality in Critically Ill Children: A Cohort Study and Systematic Review. Pediatr Crit Care Med 2016;17:e444-50. [Crossref] [PubMed]
- Numa A, Aneja H, Awad J, et al. Admission Hyperoxia Is a Risk Factor for Mortality in Pediatric Intensive Care. Pediatr Crit Care Med 2018;19:699-704. [Crossref] [PubMed]
- Raman S, Ray S, Peters MJ. Survey of Oxygen Delivery Practices in UK Paediatric Intensive Care Units. Crit Care Res Pract 2016;2016:6312970 [Crossref] [PubMed]
- Ray S, Rogers L, Raman S, et al. Liberal oxygenation in paediatric intensive care: retrospective analysis of high-resolution SpO2 data. Intensive Care Med 2017;43:146-7. [Crossref] [PubMed]
- Network NNAC. ARDSnet mechanical ventilation protocol card. Available online: http://www.ardsnet.org/tools.shtml
- Brower RG, Matthay MA, Morris A, et al. Ventilation with lower tidal volumes as compared with traditional tidal volumes for acute lung injury and the acute respiratory distress syndrome. N Engl J Med 2000;342:1301-8. [Crossref] [PubMed]
- Kneyber MCJ, de Luca D, Calderini E, et al. Recommendations for mechanical ventilation of critically ill children from the Paediatric Mechanical Ventilation Consensus Conference (PEMVECC). Intensive Care Med 2017;43:1764-80. [Crossref] [PubMed]
- Elmer J, Scutella M, Pullalarevu R, et al. The association between hyperoxia and patient outcomes after cardiac arrest: analysis of a high-resolution database. Intensive Care Med 2015;41:49-57. [Crossref] [PubMed]
- Kilgannon JH, Jones AE, Parrillo JE, et al. Relationship between supranormal oxygen tension and outcome after resuscitation from cardiac arrest. Circulation 2011;123:2717-22. [Crossref] [PubMed]
- Rincon F, Kang J, Maltenfort M, et al. Association between hyperoxia and mortality after stroke: a multicenter cohort study. Crit Care Med 2014;42:387-96. [Crossref] [PubMed]
- de Jonge E, Peelen L, Keijzers PJ, et al. Association between administered oxygen, arterial partial oxygen pressure and mortality in mechanically ventilated intensive care unit patients. Crit Care 2008;12:R156. [Crossref] [PubMed]
- Chu DK, Kim LH, Young PJ, et al. Mortality and morbidity in acutely ill adults treated with liberal versus conservative oxygen therapy (IOTA): a systematic review and meta-analysis. Lancet 2018;391:1693-705. [Crossref] [PubMed]
- Siemieniuk RAC, Chu DK, Kim LH, et al. Oxygen therapy for acutely ill medical patients: a clinical practice guideline. BMJ 2018;363:k4169. [Crossref] [PubMed]
- Sznycer-Taub NR, Lowery R, Yu S, et al. Hyperoxia Is Associated With Poor Outcomes in Pediatric Cardiac Patients Supported on Venoarterial Extracorporeal Membrane Oxygenation. Pediatr Crit Care Med 2016;17:350-8. [Crossref] [PubMed]
- Cunningham S, Rodriguez A, Adams T, et al. Oxygen saturation targets in infants with bronchiolitis (BIDS): a double-blind, randomised, equivalence trial. Lancet 2015;386:1041-8. [Crossref] [PubMed]
- Askie LM, Darlow BA, Davis PG, et al. Effects of targeting lower versus higher arterial oxygen saturations on death or disability in preterm infants. Cochrane Database Syst Rev 2017;4:Cd011190 [PubMed]
- Fleming S, Thompson M, Stevens R, et al. Normal ranges of heart rate and respiratory rate in children from birth to 18 years of age: a systematic review of observational studies. Lancet 2011;377:1011-8. [Crossref] [PubMed]
- Bonafide CP, Brady PW, Keren R, et al. Development of heart and respiratory rate percentile curves for hospitalized children. Pediatrics 2013;131:e1150-7. [Crossref] [PubMed]
- Daymont C, Bonafide CP, Brady PW. Heart rates in hospitalized children by age and body temperature. Pediatrics 2015;135:e1173-81. [Crossref] [PubMed]
- Davis AL, Carcillo JA, Aneja RK, et al. The American College of Critical Care Medicine Clinical Practice Parameters for Hemodynamic Support of Pediatric and Neonatal Septic Shock: Executive Summary. Pediatr Crit Care Med 2017;18:884-90. [Crossref] [PubMed]
- Pollack MM, Ruttimann UE, Getson PR. Pediatric risk of mortality (PRISM) score. Crit Care Med 1988;16:1110-6. [Crossref] [PubMed]
- Griffin MP, Lake DE, Bissonette EA, et al. Heart rate characteristics: novel physiomarkers to predict neonatal infection and death. Pediatrics 2005;116:1070-4. [Crossref] [PubMed]
- Fairchild KD. Predictive monitoring for early detection of sepsis in neonatal ICU patients. Curr Opin Pediatr 2013;25:172-9. [Crossref] [PubMed]
- Kamaleswaran R, Akbilgic O, Hallman MA, et al. Applying Artificial Intelligence to Identify Physiomarkers Predicting Severe Sepsis in the PICU. Pediatr Crit Care Med 2018;19:e495-503. [PubMed]
- Straney L, Clements A, Parslow RC, et al. Paediatric index of mortality 3: an updated model for predicting mortality in pediatric intensive care*. Pediatr Crit Care Med 2013;14:673-81. [Crossref] [PubMed]
- Pollack MM, Patel KM, Ruttimann UE. PRISM III: an updated Pediatric Risk of Mortality score. Crit Care Med 1996;24:743-52. [Crossref] [PubMed]
- Shann F, Pearson G, Slater A, et al. Paediatric index of mortality (PIM): a mortality prediction model for children in intensive care. Intensive Care Med 1997;23:201-7. [Crossref] [PubMed]
- Brierley J, Carcillo JA, Choong K, et al. Clinical practice parameters for hemodynamic support of pediatric and neonatal septic shock: 2007 update from the American College of Critical Care Medicine. Crit Care Med 2009;37:666-88. [Crossref] [PubMed]
- Farrell CA LJ. The fourth report on the diagnosis, evaluation, and treatment of high blood pressure in children and adolescents. Pediatrics 2004;114:555-76. [Crossref] [PubMed]
- Marlais M, Lyttle MD, Inwald D. Ten concerns about blood pressure measurement and targets in paediatric sepsis. Intensive Care Med 2017;43:433-5. [Crossref] [PubMed]
- Report of the Second Task Force on Blood Pressure Control in Children--1987. Task Force on Blood Pressure Control in Children. National Heart, Lung, and Blood Institute, Bethesda, Maryland. Pediatrics 1987;79:1-25. [PubMed]
- Abdelrazeq S, Ray S, Rogers L, et al. Age-associated blood pressure distributions in paediatric intensive care units differ from healthy children. Intensive Care Med 2018;44:384-6. [Crossref] [PubMed]
- Ray S, Rogers L, Noren DP, et al. Risk of over-diagnosis of hypotension in children: a comparative analysis of over 50,000 blood pressure measurements. Intensive Care Med 2017;43:1540-1. [Crossref] [PubMed]
- Topjian AA, French B, Sutton RM, et al. Early postresuscitation hypotension is associated with increased mortality following pediatric cardiac arrest. Crit Care Med 2014;42:1518-23. [Crossref] [PubMed]
- Topjian AA, Telford R, Holubkov R, et al. Association of Early Postresuscitation Hypotension With Survival to Discharge After Targeted Temperature Management for Pediatric Out-of-Hospital Cardiac Arrest: Secondary Analysis of a Randomized Clinical Trial. JAMA Pediatr 2018;172:143-53. [Crossref] [PubMed]
- Lacroix J, Tucci M, Du Pont-Thibodeau G. Red blood cell transfusion decision making in critically ill children. Curr Opin Pediatr 2015;27:286-91. [Crossref] [PubMed]
- Valentine SL, Bembea MM, Muszynski JA, et al. Consensus Recommendations for RBC Transfusion Practice in Critically Ill Children From the Pediatric Critical Care Transfusion and Anemia Expertise Initiative. Pediatr Crit Care Med 2018;19:884-98. [Crossref] [PubMed]
- D'Alessandro A, Kriebardis AG, Rinalducci S, et al. An update on red blood cell storage lesions, as gleaned through biochemistry and omics technologies. Transfusion 2015;55:205-19. [Crossref] [PubMed]
- Bennett-Guerrero E, Veldman TH, Doctor A, et al. Evolution of adverse changes in stored RBCs. Proc Natl Acad Sci U S A 2007;104:17063-8. [Crossref] [PubMed]
- Muszynski JA, Frazier E, Nofziger R, et al. Red blood cell transfusion and immune function in critically ill children: a prospective observational study. Transfusion 2015;55:766-74. [Crossref] [PubMed]
- Nellis ME, Goel R, Feinstein S, et al. Association Between Transfusion of RBCs and Subsequent Development of Delirium in Critically Ill Children. Pediatr Crit Care Med 2018;19:925-9. [PubMed]
- Lacroix J, Hebert PC, Hutchison JS, et al. Transfusion strategies for patients in pediatric intensive care units. N Engl J Med 2007;356:1609-19. [Crossref] [PubMed]
- Adams ES. (N Engl J Med 2007; 356:1609-1619). Pediatr Crit Care Med 2009;10:393-6. [Crossref] [PubMed]
- Marsh K, Forster D, Waruiru C, et al. Indicators of life-threatening malaria in African children. N Engl J Med 1995;332:1399-404. [Crossref] [PubMed]
- Bembea MM, Cheifetz IM, Fortenberry JD, et al. Recommendations on the Indications for RBC Transfusion for the Critically Ill Child Receiving Support From Extracorporeal Membrane Oxygenation, Ventricular Assist, and Renal Replacement Therapy Devices From the Pediatric Critical Care Transfusion and Anemia Expertise Initiative. Pediatr Crit Care Med 2018;19:S157-62. [Crossref] [PubMed]
- Cook JL, Colvin M, Francis GS, et al. Recommendations for the Use of Mechanical Circulatory Support: Ambulatory and Community Patient Care: A Scientific Statement From the American Heart Association. Circulation 2017;135:e1145-58. [Crossref] [PubMed]
- Curley A, Stanworth SJ, Willoughby K, et al. Randomized Trial of Platelet-Transfusion Thresholds in Neonates. N Engl J Med 2018; [Epub ahead of print]. [Crossref] [PubMed]
- Benedetti Md GM, Silverstein Md FS. Targeted Temperature Management in Pediatric Neurocritical Care. Pediatr Neurol 2018;88:12-24. [Crossref] [PubMed]
- Azzopardi D, Brocklehurst P, Edwards D, et al. The TOBY Study. Whole body hypothermia for the treatment of perinatal asphyxial encephalopathy: a randomised controlled trial. BMC Pediatr 2008;8:17. [Crossref] [PubMed]
- Schortgen F. Fever in sepsis. Minerva Anestesiol 2012;78:1254-64. [PubMed]
- Brick T, Agbeko RS, Davies P, et al. Attitudes towards fever amongst UK paediatric intensive care staff. Eur J Pediatr 2017;176:423-7. [Crossref] [PubMed]
- Prymula R, Siegrist CA, Chlibek R, et al. Effect of prophylactic paracetamol administration at time of vaccination on febrile reactions and antibody responses in children: two open-label, randomised controlled trials. Lancet 2009;374:1339-50. [Crossref] [PubMed]
- Doran TF, De Angelis C, Baumgardner RA, et al. Acetaminophen: more harm than good for chickenpox? J Pediatr 1989;114:1045-8. [Crossref] [PubMed]
- Brandts CH, Ndjave M, Graninger W, et al. Effect of paracetamol on parasite clearance time in Plasmodium falciparum malaria. Lancet 1997;350:704-9. [Crossref] [PubMed]
- Ray S, Brick T, Raman S, et al. Haemodynamic changes with paracetamol in critically-ill children. J Crit Care 2017;40:108-12. [Crossref] [PubMed]
- Lee BH, Inui D, Suh GY, et al. Association of body temperature and antipyretic treatments with mortality of critically ill patients with and without sepsis: multi-centered prospective observational study. Crit Care 2012;16:R33. [Crossref] [PubMed]
- Young PJ, Saxena M, Beasley R, et al. Early peak temperature and mortality in critically ill patients with or without infection. Intensive Care Med 2012; [Epub ahead of print]. [Crossref] [PubMed]
- Gordijn MS, Plotz FB, Kneyber MC. Fever during pediatric intensive care unit admission is independently associated with increased morbidity. J Intensive Care Med 2009;24:317-22. [Crossref] [PubMed]
- McIntyre J, Hull D. Metabolic rate in febrile infants. Arch Dis Child 1996;74:206-9. [Crossref] [PubMed]
- Hirshberg EL, Sward KA, Faustino EV, et al. Clinical equipoise regarding glycemic control: a survey of pediatric intensivist perceptions. Pediatr Crit Care Med 2013;14:123-9. [Crossref] [PubMed]
- Hirshberg E, Larsen G, Van Duker H. Alterations in glucose homeostasis in the pediatric intensive care unit: Hyperglycemia and glucose variability are associated with increased mortality and morbidity. Pediatr Crit Care Med 2008;9:361-6. [Crossref] [PubMed]
- Yates AR, Dyke PC 2nd, Taeed R, et al. Hyperglycemia is a marker for poor outcome in the postoperative pediatric cardiac patient. Pediatr Crit Care Med 2006;7:351-5. [Crossref] [PubMed]
- Faustino EV, Apkon M. Persistent hyperglycemia in critically ill children. J Pediatr 2005;146:30-4. [Crossref] [PubMed]
- Faustino EV, Bogue CW. Relationship between hypoglycemia and mortality in critically ill children. Pediatr Crit Care Med 2010;11:690-8. [Crossref] [PubMed]
- Faustino EV, Hirshberg EL, Bogue CW. Hypoglycemia in critically ill children. J Diabetes Sci Technol 2012;6:48-57. [Crossref] [PubMed]
- van den Berghe G, Wouters P, Weekers F, et al. Intensive insulin therapy in critically ill patients. N Engl J Med 2001;345:1359-67. [Crossref] [PubMed]
- Finfer S, Chittock DR, Su SY, et al. Intensive versus conventional glucose control in critically ill patients. N Engl J Med 2009;360:1283-97. [Crossref] [PubMed]
- Vlasselaers D, Milants I, Desmet L, et al. Intensive insulin therapy for patients in paediatric intensive care: a prospective, randomised controlled study. Lancet 2009;373:547-56. [Crossref] [PubMed]
- Agus MS, Steil GM, Wypij D, et al. Tight glycemic control versus standard care after pediatric cardiac surgery. N Engl J Med 2012;367:1208-19. [Crossref] [PubMed]
- Macrae D, Grieve R, Allen E, et al. A randomized trial of hyperglycemic control in pediatric intensive care. N Engl J Med 2014;370:107-18. [Crossref] [PubMed]
- Agus MS, Wypij D, Hirshberg EL, et al. Tight Glycemic Control in Critically Ill Children. N Engl J Med 2017;376:729-41. [Crossref] [PubMed]
- Fivez T, Kerklaan D, Mesotten D, et al. Early versus Late Parenteral Nutrition in Critically Ill Children. N Engl J Med 2016;374:1111-22. [Crossref] [PubMed]
- Aufderheide TP, Lurie KG. Death by hyperventilation: a common and life-threatening problem during cardiopulmonary resuscitation. Crit Care Med 2004;32:S345-51. [Crossref] [PubMed]
- Aufderheide TP, Sigurdsson G, Pirrallo RG, et al. Hyperventilation-induced hypotension during cardiopulmonary resuscitation. Circulation 2004;109:1960-5. [Crossref] [PubMed]
- Vissers G, Soar J, Monsieurs KG. Ventilation rate in adults with a tracheal tube during cardiopulmonary resuscitation: A systematic review. Resuscitation 2017;119:5-12. [Crossref] [PubMed]
- Benoit DD, Jensen HI, Malmgren J, et al. Outcome in patients perceived as receiving excessive care across different ethical climates: a prospective study in 68 intensive care units in Europe and the USA. Intensive Care Med 2018;44:1039-49. [Crossref] [PubMed]
Cite this article as: Feinstein Y, Matettore A, Ray S, Brown K, Ramnarayan P, Peters M. Limitations of adaptive physiology: are normal values really normal in the critically ill child? Pediatr Med 2019;2:1.