Energy expenditure in critically ill children
Introduction
In critically ill children, adequate energy intake is associated with improved clinical outcome (1-3). The determination of energy requirements throughout stay in the pediatric intensive care unit (PICU) is challenging and both underfeeding and overfeeding have to be avoided. Energy requirements are depending on the different phases of illness (i.e., the acute, stable and recovery phase) and may be affected by the severity of illness and by factors such as sedation, muscle relaxants, mechanical ventilation and fever. To determine the energy requirements of critically ill children, resting energy expenditure (REE) is measured with indirect calorimetry (IC) or otherwise calculated using a predictive equation, and if needed, disease and activity factors are considered.
Measurements of REE by IC in critically ill children
Components of total energy expenditure (TEE) in healthy children
In healthy children, the TEE expended by children over 24 hours is made up by the basal metabolic rate (BMR), the thermic effect of food, physical activity, growth and, rarely, cold-induced thermogenesis (4). The BMR is the main component of TEE, and can be considered as the sum of the energy expenditure of the various organs and tissues of the body (4,5). BMR—expressed in kcal/kg of body weight per day—is high in infants and young children due to their body composition with a relatively large proportion of organs with high metabolic rate. The energy needed for growth results from energy expenditure of protein and lipid synthesis and energy deposition in newly formed tissues (4). This expenditure is particularly important in rapidly growing infants (approximately up to the age of 12 months) and during puberty (6). As a result, TEE is high in young children in comparison to older children and adults, as illustrated in Figure 1.
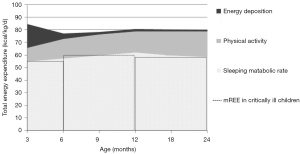
Precise measurement of BMR requires very strict conditions i.e. fasting for at least 12–14 hours, being awake, supine, resting comfortably, not having done strenuous exercise in the preceding day, etc. (4). These conditions are rarely achieved. Therefore, most studies measure the REE—the energy expended when the body is at rest, with no extra energy used for muscular effort (4). REE is usually slightly higher (up to 10%) than BMR and is often used as a proxy for BMR.
Main principles of measurement of REE with IC
For measurement of the REE in clinical practice, IC remains the reference method. The principle of IC is that energy production by substrate oxidation in the body is coupled to oxygen consumption (VO2) and carbon dioxide production (VCO2) (8). The ratio between VCO2 and VO2 associated with metabolic processes at the cellular level is called the respiratory quotient (RQ): RQ = VCO2/VO2 (8). The RQ and the energy released depend on the particular substrate that is oxidized, as described in Table 1. For instance, the stoichiometric equation for the oxidation of 100 gram of glucose is as follows (8):
Table 1
Substrate | RQ | Caloric value (kcal/g) | O2 consumption (L/g) | CO2 production (L/g) |
---|---|---|---|---|
Glucose | 1.00 | 4.18 | 0.829 | 0.829 |
Protein | 0.81 | 4.32 | 0.966 | 0.782 |
Fat | 0.71 | 9.46 | 2.019 | 1.427 |
To calculate REE from VO2 and VCO2, various formulas have been developed. The Weir formula is the most commonly used (9):
IC measures REE over a brief period (30 minutes–two hours) under strict conditions, and this value is mostly used for the REE over 24 hours.
Practical use of IC measurement in PICU
To determine energy expenditure in critically ill children and guide nutritional support, the European Society of Pediatric and Neonatal Intensive Care (ESPNIC) and the American Society for Parenteral and Enteral Nutrition (A.S.P.E.N) recommend to measure REE, using a validated indirect calorimeter, after the acute phase of critical illness (10,11). For targeting patients who could be a candidate for IC measurement, a list of 11 criteria has been proposed (Table 2) (12). It has been shown that according to these criteria, more than 70% of children admitted to the PICU would require IC measurement (13).
Table 2
Underweight (BMI <5th percentile for age), at risk of overweight (BMI >85th percentile for age) or overweight (BMI >95th percentile for age) |
Children with >10% weight gain or loss during ICU stay |
Failure to consistently meet prescribed caloric goals |
Failure to wean, or need to escalate respiratory support |
Need for muscle relaxants for >7 days |
Neurologic trauma (traumatic, hypoxic and/or ischemic) with evidence of dysautonomia |
Oncologic diagnoses (including children with stem cell or bone marrow transplant) |
Children with thermal injury |
Children requiring mechanical ventilator support for >7 days |
Children suspected to be severely hypermetabolic (status epilepticus, hyperthermia, systemic inflammatory response syndrome, dysautonomic storms, etc.) or hypometabolic (hypothermia, hypothyroidism, pentobarbital or midazolam coma, etc.) |
Any patient with ICU LOS >4 weeks may benefit from IC to assess adequacy of nutrient intake |
IC, indirect calorimetry; PICU, pediatric intensive care unit.
Measuring REE with IC requires financial, technical and human resources, which may explain why only a small minority of PICUs make use of an indirect calorimeter (10–17% of PICUs worldwide) (1,14-16). In ventilated patients, some conditions such as high inspired oxygen concentrations, potential leaks around the endotracheal tube or humidity may lead to inaccurate measurements. Clinicians must ensure that conditions for accurate calibration of the device and valid measurement at bedside are achieved. Until recently, the majority of PICUs used the Deltatrac II, (Datex-Ohmeda, Helsinki, Finland), which has been validated in critically ill adults and children (17-19). However, this device is no longer being manufactured. Currently, among the available indirect calorimeters used in adults (M-COVX, Datex-Ohmeda, Finland; Quark RMR and Q-NRG+, Cosmed, Italy, and CCM Express, Medical Graphics Corp, UK), none has been validated in ventilated critically ill children. Thus, a validated calorimeter is urgently needed for the pediatric population including new-born and infants and those with high inspired oxygen concentrations (up to 60–70%).
Estimation of REE by predictive equations in critically ill children
Available predictive equations in healthy children
As an alternative for REE measurement with IC, predictive equations can be used to estimate REE. Various equations exist and most of them are based on age, sex, body weight and height. To estimate REE in critically ill children, international nutritional guidelines (10,11,20) recommend to estimate REE using the Schofield equation, with an accurate weight (21). Table 3 shows the Schofield equation for boys and girls, divided by different age categories.
Table 3
Age (years) | Girls | Boys |
---|---|---|
<3 | 58.317 × W − 31.1 | 59.512 × W − 30.4 |
3–10 | 20.315 × W + 485.9 | 22.706 × W + 504.3 |
10–18 | 13.384 × W + 692.6 | 17.686 × W + 658.2 |
W = weight in kg.
Validity of the commonly used predictive equations of REE in critically ill children
According to a worldwide survey (15), most PICUs estimate REE using the common predictive equations of Schofield (25%) (21), the equation of the World Health Organization (25%) (5) and, more surprisingly, the equation of Harris-Benedict (17%) (22).
The validity of Schofield equations (21) has been evaluated in a number of studies in critically ill children (23). The findings showed that the two Schofield equations [along with Talbot tables (24)] compared with many other equations were the least inaccurate (23). However, prediction of REE within ±10% compared to REE measured by IC (mREE) was only noticed in about 35% of children. These equations may underestimate REE in young children and overestimate it in older children (25).
Nutritional guidelines (10,11) recommend not using the Harris-Benedict equation (22) in critically ill children, as there is strong evidence that this equation, developed for healthy adults, overestimates REE in the large majority of children and leads to a risk of overfeeding.
Validity of specific equations developed to estimate REE in ventilated critically ill children
Some equations have been designed for populations with specific diseases or conditions including ventilated critically ill children; namely the equations of White et al. in 2000 (26), Meyer et al. in 2012 (27) and Mehta et al. in 2015 (28). A few studies have assessed the validity of the equations of White (26) and Meyer (27), and showed that these equations were inaccurate (23,25,29). The equation developed by Mehta et al. (28) in 2015 seems promising. This equation is not a traditional equation, as it requires measurement of VCO2 and it uses a fixed RQ of 0.89 in the Weir formula (9). A recent study conducted in children after cardiopulmonary bypass showed that the most important determinant of its bias was the RQ (30). Another group demonstrated that estimating REE with this equation, using VCO2 measured by the Servo-I® with the Capnostat-III sensor, was accurate for children weighing more than 15 kg, but not for smaller children (31). The main problem in children weighing less than 15 kg was that the used sensor did not measure the VCO2 accurately enough. Thus, future studies need to assess the validity of this equation, and in parallel, a sensor for children weighing less than 15 kg needs to be developed.
Influence of critical care conditions on REE in critically ill children
Energy expenditure in critically ill children
Critically ill patients are lying in bed in a thermoneutral environment, are not physically active, sedated and regularly mechanically ventilated. Therefore, energy expenditure is lower than in healthy children (Figure 1) and it is often assumed that TEE is equal to REE in the acute phase of disease. Several studies, with the large majority conducted in children on mechanical ventilation, have investigated which factors during intensive care treatment might influence individual energy expenditure (Table 4).
Table 4
Factor | Increase or decrease | Percentage change in relation to mREE | References |
---|---|---|---|
Physical activity | ↑ | 20% | (32) |
Body temperature, per °C | ↑ | Up to 8% | (7,29,33,34) |
Sedatives | ↓/– | – | (29,35,36) |
Muscle relaxants | ↓/– | – | (7,29,35,37-40) |
Specific diseases | |||
Surgery | – | – | (41-46) |
↑ | Up to 15%, post-admission in infants | (47-49) | |
Sepsis | – | – | (32,36,50-52) |
↑ | Up to 40% during the recovery phase in neonates | (52,53) | |
Traumatic brain injury | ↑/– | – | (34,54-56) |
Trauma | ↑/↓ | – | (54,57,58) |
Burns, acute phase | ↑ | Up to 80% | (59-63) |
Burns, recovery phase | ↑ | Up to 15% | (59,62,63) |
↑ increase, ↓ decrease, – no change. REE, resting energy expenditure.
Day-to-day variability of REE in mechanically ventilated children
In mechanically ventilated children with various medical and surgical diagnoses, no clinically significant day-to-day changes in mREE were observed in the first week after PICU admission (7,37,38,50,51,64). This implies that intra-individually, mREE does not change significantly during this period on mechanical ventilation.
Body temperature
Body temperature has been found to be the most important factor to influence mREE within the individual patient (26). In a study of 74 critically ill, mechanically ventilated children, every increment of degree Celsius increased mREE by 8% (7). Other studies have also shown this positive correlation with factors per degree Celsius ranging from 6% to 8% (29,33,34).
Drugs
Sedatives and vasoactive drugs are frequently used in critically ill children and have been reported to influence mREE (29,37). In a study of 57 mechanically ventilated children aged 9 months to 15.8 years, mREE was lower than REE estimated by predictive equation in 82% of children when sedation was used (29). However, these results were not reported in other studies (35,36). In a few studies of mechanically ventilated children, the use of muscle relaxants decreased mREE from 6% to 10% (7,29,39) and in one study even up to 36% (35). In some other studies, the use of muscle relaxants did not influence mREE (37,38,40).
Diet induced thermogenesis
It has been postulated that the amount and manner of nutritional support influences energy expenditure in critically ill patients, however no effect has been shown in studies so far (29,35). This might be due to the use of continuous nutritional support in many studies.
Physical activity
In one study of seven critically ill children, TEE was measured with the double labeled water method during six days at the PICU (32). TEE was 20% higher than REE. This increased energy expenditure was related with activity recorded with accelerometry (32). This means that physical activity levels should be accounted for in calculating total energy requirements in critically ill children who stay at least one week at the PICU.
Specific diseases
Surgery
In infants admitted in PICU after surgery, a few studies have found an increase in mREE immediately after surgery with a peak at 4 hours for up to 15% (47-49). This increase was only temporarily (47-49) and mREE returned rapidly to levels comparable to preoperatively (41-43). In studies including older children, mREE did not increase after surgery (44-46).
Sepsis
In a study of ten spontaneously breathing septic neonates, an increase of 20% in mREE (mean value of 57±3 kcal/kg per day) was found on days 1–3 after the onset of sepsis compared with healthy controls and 15% on day 4 (53). In another study of 19 septic neonates, mREE increased from 49±13 kcal/kg per day during the acute phase to 68±11 kcal/kg per day during the recovery phase of sepsis (52). According to the authors, this was probably due to the resumption of growth. In older children with sepsis, mREE was not different compared to critically ill children with other diagnoses or healthy controls (32,36,50,51).
Traumatic brain injury
Although two studies in the eighties have found increased mREE of up to two times predicted REE in children after severe traumatic brain injury (54,55), more recent studies did not show such hypermetabolic state (34,56). The authors of the latter studies suggested that this might be due to differences in treatment, as guidelines have evolved over the years concerning sedation, paralysis and temperate control of these patients. In this group, REE might be dependent on the phase of disease and the neurologic state of the individual patient.
Trauma
Only a few studies have measured REE in children after severe trauma in different populations and found both decreased and increased mREE (54,57,58).
Burns
A recent systematic review in critically ill adults and children has concluded that percentage of body surface area burn and post burn day were both associated with mREE (65). Some studies observed an increase of mREE in children with burns for up to 180% compared to predicted REE in the acute phase (59-63). Moreover, although REE decreased upon recovery, mREE remained significantly higher (up to 15%) compared to predicted REE up to months and even years postburn (59,62,63). This hypermetabolic state is considered to be mediated by increased pro-inflammatory cytokines (61,66,67), catecholamines and stress hormones (61,62) in burn patients.
In conclusion, these studies show that the main factors that may influence REE in critically ill children are body temperature and physical activity. Moreover, burns may have a significant clinical impact on energy expenditure.
Optimal energy intake in different phases of disease using REE
Energy requirements in the acute and stable phases of critical illness
The acute phase is characterized by the requirement of vital organ support and is accompanied by a stress response, resulting in hypercatabolism (68). The pathways of energy production during the acute phase of critical illness are altered and alternative substrates are used as a result of the loss of control of energy substrate utilization by their availability. Traditionally, overfeeding and underfeeding were defined as the percentage of energy delivery related to the mREE; an intake of >110% mREE indicated overfeeding and an intake of <90% mREE indicated underfeeding (69). However, during the acute phase of critical illness, endogenous production of energy provides the majority of energy requirements (up to 75%), irrespective of the exogenous provided amount of energy (Figure 2) (71). This results in a considerable risk for an energy imbalance, which is associated with poor outcome.
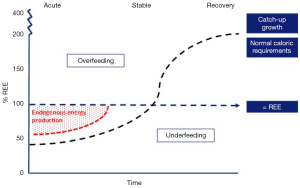
In a recent retrospective study, patients that were overfed (>110% mREE) had a significant longer PICU and hospital stays compared to those who were adequately fed (90–110% mREE) (72). Moreover, in a large multicenter randomized controlled trial it was shown that withholding parenteral nutrition (PN) until day 8 of admission, as compared with starting PN early (<24 hours) when enteral nutrition (EN) was insufficient, and thereby accepting significantly lower energy intakes compared to predicted REE, significantly decreased nosocomial infection and accelerated recovery (2).
New PICU guidelines advocate that in the acute phase of critical illness energy intake should not exceed REE (10,11). Furthermore, it is recommended to increase EN in a stepwise fashion until delivery goal is achieved using a feeding protocol or guideline and to target an energy intake by the end of the first week in the PICU of at least two-thirds of the daily energy requirements (Table 5) (10,11). These guidelines are based on observational studies that showed better clinical outcomes in patients who received adequate nutritional intake (1,3,72). However, not all of these studies measured REE. At this stage, no interventional studies determined the precise energy intake related to improved outcomes in critically ill children. In a systematic review (73), followed by an observational study (7), it was observed that a minimum intake of 57 kcal/kg per day (and 1.5 g/kg per day of protein) was associated with positive nitrogen balance.
Table 5
Phase | Enteral nutrition | Parenteral nutrition | Energy target |
---|---|---|---|
Acute phase | Increase EN in stepwise manner | No PN | Do not exceed 100% REE |
Stable phase | Increase EN further, if possible | Start PN >1 week after PICU admission, if energy targets are not met | Increase of %REE depending on age and disease |
Recovery phase | Increase EN further, if possible | Continue PN if necessary to achieve energy targets | Up to 200% REE (and occasionally up to 400% REE). Target for (catch-up) growth |
EN, enteral nutrition; PN, parenteral nutrition; REE, resting energy expenditure.
Energy requirements during the recovery phase of critical illness
The recovery phase starts when the patient is mobilized and the stress response is resolving and normalized. This phase could last up to several months. During this phase, the body shifts further from catabolism to anabolism with protein synthesis exceeding protein breakdown, which results in tissue repair and (catch-up) growth (68). Thereby, energy requirements may rise considerably, even exceeding normal energy requirements of healthy children (Figure 2) (70,74). Recent guidelines state that, after the acute phase, energy intake should account for energy debt, physical activity, rehabilitation and growth (Table 5) (10). Only a few studies have investigated the energy requirements in the recovery phase, mostly in children with heart disease and burns. Recently, it was shown in critically ill infants mainly with heart disease and with a mean PICU stay of 50 days that weight gain was achieved comparable with healthy children by following a nutritional protocol with energy target set at 2× predicted REE (75). In infants recovering from surgical repair of congenital heart disease, caloric goals of 2–3× REE (120–150 kcal/kg/day) are presumed to be necessary for weight gain and even up to 4× REE (200 kcal/kg/day) for infants with remained hemodynamically significant lesions (76,77). In burned patients, a hypermetabolic state with increased nutritional demands has been shown even up to two years after injury (59,62,78). One study, published in 1988, showed that energy intake should be approximately 1.6× REE to maintain weight in this group (79).
The use of the RQ
The RQ may help evaluate substrate utilization and/or nutritional support, and determine overfeeding and underfeeding. As shown in Table 1, fat oxidation results in an RQ of 0.7, whereas protein and carbohydrate oxidation result in RQs of 0.8 and 1.0, respectively. Carbohydrate intake higher than oxidative capacity may result in lipogenesis, leading to an RQ >1.0, which makes it a possible marker for detecting overfeeding (80). Moreover, the use of endogenous fat stores to meet energy requirements can decrease the RQ below 0.85 (59). However, cut-off values for the RQ (<0.85, 0.85–1.0, >1.0) correspond only moderately with the currently used criteria of under- and overfeeding (the ratio of intake and mREE) (81,82). Alternatively, the use of a difference between measured RQ and predicted RQ [using the proportions of carbohydrates and fats in nutritional intake (RQmacr)], of more than >0.05 has been proposed as a definition of overfeeding (83). This definition might be a more useful marker, as the RQ can alter during PICU admission due to change in phase of disease. Hence, the RQ can be used to determine under- and overfeeding and to monitor the tolerance of nutritional support. However, the RQ cannot be used to “fine-tune” nutritional therapy because each patient has his/her own metabolic “fingerprint” or “signature” inheriting a unique metabolic machinery capable of generating a particular, and not always predictable, response during the different phase of the stress response.
Overall, measurements of REE and RQ after the acute phase of disease may be helpful to guide nutritional therapy and to increase nutritional intake in relation to REE until (catch-up) growth and tissue repair are achieved.
Conclusions
In critically ill children, clinicians have the complex role of prescribing an optimal energy intake, avoiding both underfeeding and overfeeding. During the acute phase, energy intake should be increased progressively according to the tolerance of the patients and not exceed REE, predicted by Schofield equation (21). Afterwards, energy intake may be increased considering the energy debt, physical activity, rehabilitation and growth. Usually this means that the target energy intake should be between 100% and 200% of REE. In some patients, measurement of REE using a validated calorimeter may be needed. In addition to measured REE, the RQ might be a helpful tool as well. The presence of a nutrition team including a dedicated dietitian is recommended to guide energy intake and nutritional support.
Future studies need to validate a new calorimeter for this specific population, including neonates and infants and patients on high inspired oxygen concentrations. In parallel, the development of more accurate predictive equations of REE or other methods to measure energy expenditure is needed.
Acknowledgments
Funding: None.
Footnote
Provenance and Peer Review: This article was commissioned by the Guest Editors (Lyvonne Tume, Frederic Valla and Sascha Verbruggen) for the series “Nutrition in the Critically Ill Child” published in Pediatric Medicine. The article was sent for external peer review organized by the Guest Editors and the editorial office.
Conflicts of Interest: The authors have completed the ICMJE uniform disclosure form (available at http://dx.doi.org/10.21037/pm-20-62). The series “Nutrition in the Critically Ill Child” was commissioned by the editorial office without any funding or sponsorship. CJC reports non-financial support from Baxter and non-financial support from Nutricia, outside the submitted work. The authors have no other conflicts of interest to declare.
Ethical Statement: The authors are accountable for all aspects of the work in ensuring that questions related to the accuracy or integrity of any part of the work are appropriately investigated and resolved.
Open Access Statement: This is an Open Access article distributed in accordance with the Creative Commons Attribution-NonCommercial-NoDerivs 4.0 International License (CC BY-NC-ND 4.0), which permits the non-commercial replication and distribution of the article with the strict proviso that no changes or edits are made and the original work is properly cited (including links to both the formal publication through the relevant DOI and the license). See: https://creativecommons.org/licenses/by-nc-nd/4.0/.
References
- Mehta NM, Bechard LJ, Cahill N, et al. Nutritional practices and their relationship to clinical outcomes in critically ill children--an international multicenter cohort study. Crit Care Med 2012;40:2204-11. [Crossref] [PubMed]
- Fivez T, Kerklaan D, Mesotten D, et al. Early versus Late parenteral nutrition in critically ill children. New Engl J Med 2016;374:1111-22. [Crossref] [PubMed]
- Wong JJ, Han WM, Sultana R, et al. Nutrition Delivery Affects Outcomes in Pediatric Acute Respiratory Distress Syndrome. JPEN J Parenter Enteral Nutr 2017;41:1007-13. [Crossref] [PubMed]
- European Food Safety Authority. Scientific Opinion on Dietary Reference Values for energy. EFSA J 2013;11:3005. [Crossref]
- FAO/WHO/UNU. Human energy requirements :report of a Joint FAO/WHO/UNU Expert Consultation: Rome, 17-24 October 2001.
- Butte NF, Wong WW, Hopkinson JM, et al. Energy requirements derived from total energy expenditure and energy deposition during the first 2 y of life. Am J Clin Nutr 2000;72:1558-69. [Crossref] [PubMed]
- Jotterand Chaparro C, Laure Depeyre J, Longchamp D, et al. How much protein and energy are needed to equilibrate nitrogen and energy balances in ventilated critically ill children? Clin Nutr 2016;35:460-7. [Crossref] [PubMed]
- Takala J, Meriläinen P. Handbook of gas exchange and indirect calorimetry. Helsinki: Datex, 1991.
- Weir JB. New methods for calculating metabolic rate with special reference to protein metabolism. J Physiol 1949;109:1-9. [Crossref] [PubMed]
- Tume LN, Valla FV, Joosten K, et al. Nutritional support for children during critical illness: European Society of Pediatric and Neonatal Intensive Care (ESPNIC) metabolism, endocrine and nutrition section position statement and clinical recommendations. Intensive Care Med 2020;46:411-25. [Crossref] [PubMed]
- Mehta NM, Skillman HE, Irving SY, et al. Guidelines for the Provision and Assessment of Nutrition Support Therapy in the Pediatric Critically Ill Patient: Society of Critical Care Medicine and American Society for Parenteral and Enteral Nutrition. Pediatr Crit Care Med 2017;18:675-715. [Crossref] [PubMed]
- Mehta NM, Compher C A.S.P.E.N.. Clinical Guidelines: nutrition support of the critically ill child. JPEN J Parenter Enteral Nutr 2009;33:260-76. [Crossref] [PubMed]
- Kyle UG, Arriaza A, Esposito M, et al. Is indirect calorimetry a necessity or a luxury in the pediatric intensive care unit? JPEN J Parenter Enteral Nutr 2012;36:177-82. [Crossref] [PubMed]
- van der Kuip M, Oosterveld MJ. Nutritional support in 111 pediatric intensive care units: a European survey. Intensive Care Med 2004;30:1807-13. [Crossref] [PubMed]
- Kerklaan D, Fivez T, Mehta NM, et al. Worldwide Survey of Nutritional Practices in PICUs. Pediatr Crit Care Med 2016;17:10-8. [Crossref] [PubMed]
- Valla FV, Gaillard-Le Roux B, Ford-Chessel C, et al. A nursing survey on nutritional care practices in French-speaking pediatric intensive care units: NutriRéa-ped 2014. J Pediatr Gastroenterol Nutr 2016;62:174-9. [Crossref] [PubMed]
- Takala J, Keinanen O, Vaisanen P, et al. Measurement of gas exchange in intensive care: laboratory and clinical validation of a new device. Crit Care Med 1989;17:1041-7. [Crossref] [PubMed]
- Tissot S, Delafosse B, Bertrand O, et al. Clinical validation of the Deltatrac monitoring system in mechanically ventilated patients. Intensive Care Med 1995;21:149-53. [Crossref] [PubMed]
- Weyland W, Weyland A, Fritz U, et al. A new paediatric metabolic monitor. Intensive Care Med 1994;20:51-7. [Crossref] [PubMed]
- Mihatsch WA, Braegger C, Bronsky J, et al. ESPGHAN/ESPEN/ESPR/CSPEN guidelines on pediatric parenteral nutrition. Clin Nutr 2018;37:2303-5. [Crossref] [PubMed]
- Schofield WN. Predicting basal metabolic rate, new standards and review of previous work. Hum Nutr Clin Nutr 1985;39:5-41. [PubMed]
- Harris JA, Benedict GF. A biometric study of basal metabolism in man. Washington: Carnegie Institution of Washington, 1919.
- Jotterand Chaparro C, Moullet C, Taffe P, et al. Estimation of Resting Energy Expenditure Using Predictive Equations in Critically Ill Children: Results of a Systematic Review. JPEN J Parenter Enteral Nutr 2018;42:976-86. [Crossref] [PubMed]
- Talbot FB. Basal metabolism standards for children. AM J Child 1938;55:455.
- Jotterand Chaparro C, Taffe P, Moullet C, et al. Performance of Predictive Equations Specifically Developed to Estimate Resting Energy Expenditure in Ventilated Critically Ill Children. J Pediatr 2017;184:220-6.e5. [Crossref] [PubMed]
- White MS, Shepherd RW, McEniery JA. Energy expenditure in 100 ventilated, critically ill children: Improving the accuracy of predictive equations. Crit Care Med 2000;28:2307-12. [Crossref] [PubMed]
- Meyer R, Kulinskaya E, Briassoulis G, et al. The Challenge of Developing a New Predictive Formula to Estimate Energy Requirements in Ventilated Critically Ill Children. Nutr Clin Pract 2012;27:669-76. [Crossref] [PubMed]
- Mehta NM, Smallwood CD, Joosten KF, et al. Accuracy of a simplified equation for energy expenditure based on bedside volumetric carbon dioxide elimination measurement--a two-center study. Clin Nutr 2015;34:151-5. [Crossref] [PubMed]
- Taylor RM, Cheeseman P, Preedy V, et al. Can energy expenditure be predicted in critically ill children? Pediatr Crit Care Med 2003;4:176-80. [Crossref] [PubMed]
- Mouzaki M, Schwartz SM, Mtaweh H, et al. Can Vco2-Based Estimates of Resting Energy Expenditure Replace the Need for Indirect Calorimetry in Critically Ill Children? JPEN J Parenter Enteral Nutr 2017;41:619-24. [Crossref] [PubMed]
- Kerklaan D, Augustus ME, Hulst JM, et al. Validation of ventilator-derived VCO2 measurements to determine energy expenditure in ventilated critically ill children. Clin Nutr 2017;36:452-7. [Crossref] [PubMed]
- van der Kuip M, de Meer K, Westerterp KR, et al. Physical activity as a determinant of total energy expenditure in critically ill children. Clin Nutr 2007;26:744-51. [Crossref] [PubMed]
- Joosten KF, Verhoeven JJ, Hop WC, et al. Indirect calorimetry in mechanically ventilated infants and children: accuracy of total daily energy expenditure with 2 hour measurements. Clin Nutr 1999;18:149-52. [Crossref] [PubMed]
- Matthews DS, Bullock RE, Matthews JN, et al. Temperature response to severe head injury and the effect on body energy expenditure and cerebral oxygen consumption. Arch Dis Child 1995;72:507-15. [Crossref] [PubMed]
- Coss-Bu JA, Jefferson LS, Walding D, et al. Resting energy expenditure in children in a pediatric intensive care unit: comparison of Harris-Benedict and Talbot predictions with indirect calorimetry values. Am J Clin Nutr 1998;67:74-80. [Crossref] [PubMed]
- Ismail J, Bansal A, Jayashree M, et al. Energy Balance in Critically Ill Children With Severe Sepsis Using Indirect Calorimetry: A Prospective Cohort Study. J Pediatr Gastroenterol Nutr 2019;68:868-73. [Crossref] [PubMed]
- Briassoulis G, Venkataraman S, Thompson AE. Energy expenditure in critically ill children. Crit Care Med 2000;28:1166-72. [Crossref] [PubMed]
- Gebara BM, Gelmini M, Sarnaik A. Oxygen consumption, energy expenditure, and substrate utilization after cardiac surgery in children. Crit Care Med 1992;20:1550-4. [Crossref] [PubMed]
- Vernon DD, Witte MK. Effect of neuromuscular blockade on oxygen consumption and energy expenditure in sedated, mechanically ventilated children. Crit Care Med 2000;28:1569-71. [Crossref] [PubMed]
- Lemson J, Driessen JJ, van der Hoeven JG. The effect of neuromuscular blockade on oxygen consumption in sedated and mechanically ventilated pediatric patients after cardiac surgery. Intensive Care Med 2008;34:2268-72. [Crossref] [PubMed]
- Cai W, Tao Y, Tang Q, et al. Study on energy metabolism in perioperative infants. Eur J Pediatr Surg 2006;16:227-30. [Crossref] [PubMed]
- Shanbhogue RL, Jackson M, Lloyd DA. Operation does not increase resting energy expenditure in the neonate. J Pediatr Surg 1991;26:578-80. [Crossref] [PubMed]
- Shanbhogue RL, Lloyd DA. Absence of hypermetabolism after operation in the newborn infant. JPEN J Parenter Enteral Nutr 1992;16:333-6. [Crossref] [PubMed]
- Groner JI, Brown MF, Stallings VA, et al. Resting energy expenditure in children following major operative procedures. J Pediatr Surg 1989;24:825-7; discussion 827-8. [Crossref] [PubMed]
- Powis MR, Smith K, Rennie M, et al. Effect of major abdominal operations on energy and protein metabolism in infants and children. J Pediatr Surg 1998;33:49-53. [Crossref] [PubMed]
- Botrán M, Lopez-Herce J, Mencia S, et al. Relationship between energy expenditure, nutritional status and clinical severity before starting enteral nutrition in critically ill children. Br J Nutr 2011;105:731-7. [Crossref] [PubMed]
- Jones MO, Pierro A, Hammond P, et al. The metabolic response to operative stress in infants. J Pediatr Surg 1993;28:1258-62; discussion 1262-3. [Crossref] [PubMed]
- Jones MO, Pierro A, Hashim IA, et al. Postoperative changes in resting energy expenditure and interleukin 6 level in infants. Br J Surg 1994;81:536-8. [Crossref] [PubMed]
- Jones MO, Pierro A, Hammond P, et al. The effect of major operations on heart rate, respiratory rate, physical activity, temperature and respiratory gas exchange in infants. Eur J Pediatr Surg 1995;5:9-12. [Crossref] [PubMed]
- Oosterveld MJS, Van Der Kuip M, De Meer K, et al. Energy expenditure and balance following pediatric intensive care unit admission: A longitudinal study of critically ill children. Pediatr Crit Care Med 2006;7:147-53. [Crossref] [PubMed]
- Turi RA, Petros AJ, Eaton S, et al. Energy metabolism of infants and children with systemic inflammatory response syndrome and sepsis. Ann Surg 2001;233:581-7. [Crossref] [PubMed]
- Feferbaum R, Leone C, Siqueira AA, et al. Rest energy expenditure is decreased during the acute as compared to the recovery phase of sepsis in newborns. Nutr Metab (Lond) 2010;7:63. [Crossref] [PubMed]
- Bauer J, Hentschel R, Linderkamp O. Effect of sepsis syndrome on neonatal oxygen consumption and energy expenditure. Pediatrics 2002;110:e69 [Crossref] [PubMed]
- Phillips R, Ott L, Young B, et al. Nutritional support and measured energy expenditure of the child and adolescent with head injury. J Neurosurg 1987;67:846-51. [Crossref] [PubMed]
- Moore R, Najarian MP, Konvolinka CW. Measured energy expenditure in severe head trauma. J Trauma 1989;29:1633-6. [Crossref] [PubMed]
- Mtaweh H, Smith R, Kochanek PM, et al. Energy expenditure in children after severe traumatic brain injury. Pediatr Crit Care Med 2014;15:242-9. [Crossref] [PubMed]
- Havalad S, Quaid MA, Sapiega V. Energy expenditure in children with severe head injury: lack of agreement between measured and estimated energy expenditure. Nutr Clin Pract 2006;21:175-81. [Crossref] [PubMed]
- Tilden SJ, Watkins S, Tong TK, et al. Measured energy expenditure in pediatric intensive care patients. Am J Dis Child 1989;143:490-2. [PubMed]
- Mlcak RP, Jeschke MG, Barrow RE, et al. The influence of age and gender on resting energy expenditure in severely burned children. Ann Surg 2006;244:121-30. [Crossref] [PubMed]
- Suman OE, Mlcak RP, Chinkes DL, et al. Resting energy expenditure in severely burned children: analysis of agreement between indirect calorimetry and prediction equations using the Bland-Altman method. Burns 2006;32:335-42. [Crossref] [PubMed]
- Jeschke MG, Chinkes DL, Finnerty CC, et al. Pathophysiologic response to severe burn injury. Ann Surg 2008;248:387-401. [PubMed]
- Jeschke MG, Gauglitz GG, Kulp GA, et al. Long-term persistance of the pathophysiologic response to severe burn injury. PLoS One 2011;6:e21245 [Crossref] [PubMed]
- Hart DW, Wolf SE, Mlcak R, et al. Persistence of muscle catabolism after severe burn. Surgery 2000;128:312-9. [Crossref] [PubMed]
- de Klerk G, Hop WC, de Hoog M, et al. Serial measurements of energy expenditure in critically ill children: useful in optimizing nutritional therapy? Intensive Care Med 2002;28:1781-5. [Crossref] [PubMed]
- Mtaweh H, Soto Aguero MJ, Campbell M, et al. Systematic review of factors associated with energy expenditure in the critically ill. Clin Nutr ESPEN 2019;33:111-24. [Crossref] [PubMed]
- Jeschke MG, Barrow RE, Herndon DN. Extended hypermetabolic response of the liver in severely burned pediatric patients. Arch Surg 2004;139:641-7. [Crossref] [PubMed]
- Finnerty CC, Herndon DN, Przkora R, et al. Cytokine expression profile over time in severely burned pediatric patients. Shock 2006;26:13-9. [Crossref] [PubMed]
- Joosten KF, Kerklaan D, Verbruggen SC. Nutritional support and the role of the stress response in critically ill children. Curr Opin Clin Nutr Metab Care 2016;19:226-33. [Crossref] [PubMed]
- McClave SA, Lowen CC, Kleber MJ, et al. Are patients fed appropriately according to their caloric requirements? JPEN J Parenter Enteral Nutr 1998;22:375-81. [Crossref] [PubMed]
- Joosten KFM, Eveleens RD, Verbruggen SCAT. Nutritional support in the recovery phase of critically ill children. Curr Opin Clin Nutr Metab Care 2019;22:152-8. [Crossref] [PubMed]
- Preiser JC, van Zanten ARH, Berger MM, et al. Metabolic and nutritional support of critically ill patients: consensus and controversies. Crit Care 2015;19:35. [Crossref] [PubMed]
- Larsen BMK, Beggs MR, Leong AY, et al. Can energy intake alter clinical and hospital outcomes in PICU? Clin Nutr ESPEN 2018;24:41-6. [Crossref] [PubMed]
- Bechard LJ, Parrott JS, Mehta NM. Systematic review of the influence of energy and protein intake on protein balance in critically ill children. J Pediatr 2012;161:333-9.e1. [Crossref] [PubMed]
- Joosten K, Embleton N, Yan W, et al. ESPGHAN/ESPEN/ESPR/CSPEN guidelines on pediatric parenteral nutrition: Energy. Clin Nutr 2018;37:2309-14. [Crossref] [PubMed]
- Eveleens RD, Dungen DK, Verbruggen SC, et al. Weight improvement with the use of protein and energy enriched nutritional formula in infants with a prolonged PICU stay. J Hum Nutr Diet 2019;32:3-10. [Crossref] [PubMed]
- Medoff-Cooper B, Irving SY. Innovative strategies for feeding and nutrition in infants with congenitally malformed hearts. Cardiol Young 2009;19:90-5. [Crossref] [PubMed]
- Nicholson GT, Clabby ML, Kanter KR, et al. Caloric intake during the perioperative period and growth failure in infants with congenital heart disease. Pediatr Cardiol 2013;34:316-21. [Crossref] [PubMed]
- Hart DW, Wolf SE, Herndon DN, et al. Energy expenditure and caloric balance after burn: increased feeding leads to fat rather than lean mass accretion. Ann Surg 2002;235:152-61. [Crossref] [PubMed]
- Hildreth MA, Herndon DN, Desai MH, et al. Reassessing caloric requirements in pediatric burn patients. J Burn Care Rehabil 1988;9:616-8. [Crossref] [PubMed]
- McClave SA, Snider HL. Use of indirect calorimetry in clinical nutrition. Nutr Clin Pract 1992;7:207-21. [Crossref] [PubMed]
- Hulst JM, van Goudoever JB, Zimmermann LJ, et al. Adequate feeding and the usefulness of the respiratory quotient in critically ill children. Nutrition 2005;21:192-8. [Crossref] [PubMed]
- Dokken M, Rustøen T, Stubhaug A. Indirect calorimetry reveals that better monitoring of nutrition therapy in pediatric intensive care is needed. JPEN J Parenter Enteral Nutr 2015;39:344-52. [Crossref] [PubMed]
- Kerklaan D, Hulst JM, Verhoeven JJ, et al. Use of Indirect Calorimetry to Detect Overfeeding in Critically Ill Children: Finding the Appropriate Definition. J Pediatr Gastroenterol Nutr 2016;63:445-50. [Crossref] [PubMed]
Cite this article as: Veldscholte K, Joosten K, Jotterand Chaparro C. Energy expenditure in critically ill children. Pediatr Med 2020;3:18.